Lakes play a crucial role in the water infrastructure of delta and coastal zone societies. As by 2050 half of the global human population is expected to live, work and recreate in these environments, it is important to optimise the management of lakes. The Building with Nature approach tries to find a balance between human use of lakes and maintaining the integrity of ecosystem functioning.
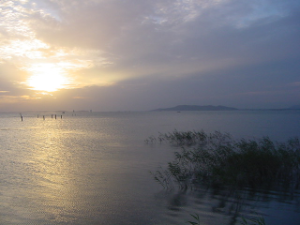
Lakes in delta and coastal areas are often formed when former lagoons or estuarine branches were partly or fully separated from tidal and salt water influences by sedimentation processes or engineering interventions (dams, gates, river training, dredging, wetland reclamation). Some lakes have a different origin, for example Lake Taihu in China and Lake Okeechobee in Florida, which originate from meteor impacts or geological processes, respectively. Lakes may also originate from excavations, e.g. of peat or clay.
Lakes that are located in low lying coastal zones and river deltas, are usually shallow. Their physical and ecological dynamics differ fundamentally from deeper stratified lakes. Often, their water has a limited residence time, as they receive water and sediments from rivers and groundwater. These exchanges determine the water balance, but also the spatial patterns in nutrient loading of the lake.
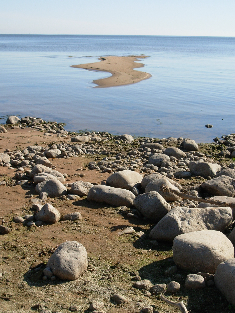
The lake ecosystem provides many ecosystem services like fresh water, habitat for fish and waterfowl, natural water purification and recreational opportunities. Many lakes occur in the vicinity of urban areas, providing important services for urban populations, e.g. Lake Pontchartrain in the Mississippi delta for New Orleans, IJsselmeer for the Randstad metropolitan area in the Netherlands and Étang de Berre for Marseille in France. Also lakes in more rural environments can be intensively used, for example Lake Peipsi in Estonia and Russia (van Eerden et al. 2007), Lake Taihu in the Yangtze delta and Songkhla Lake in Thailand.
Over the course of history, mankind has created and adapted lakes through engineering interventions for a wide range of reasons: flood control, navigation and harbour development, land reclamation, storage of fresh water. Usually, these interventions focused on the optimisation of one or few functions. Negative impacts on other functions or services would be discarded. For instance, the reclamation of wetlands was beneficial to the development of productive land, but it reduced the area of land-water gradients. This affected ecosystem services like natural water purification and the spawning of fish.
Unexpected system feedbacks led to the further reduction of ecosystem services. In many lakes, the consequences of excessive nutrient loading (eutrophication) have led to fundamental ecological changes. Eutrophied lakes are characterized by massive algal bloom and bacterial growth events, affecting the quality of fresh water supply for drinking and agriculture. Another example of an unexpected feedback is the increased vulnerability of coastal zones to flooding as wetland reclamation has reduced the flood water storage capacity.
As a result, the current state of many lakes in terms of ecology and water quality is poor and often does not comply with legal environmental standards. The ecological degradation and its consequences bring increasing management costs and challenge government budgets. Societies and their need for ecosystem services continue to grow and new ways to cope with degradation and increasing vulnerabilities have to be found. Since a few decades, the rehabilitation of lake ecosystems is becoming part of management and engineering practices.
Below we give an overview of Building with Nature opportunities for lakes. In order to have a better understanding of these opportunities, characteristics and functioning of lake ecosystems are described. Further, examples of Building with Nature interventions in lakes are presented, for example in the Lake IJssel (Dutch: IJsselmeer) region in the Netherlands. From these experiences we derive lessons that may be of use to other lakes.
System description
Lakes are generally regarded as socio-ecological systems. These are characterized by interactions between humans and the lake ecosystem. On the one hand, riparian societies adapt a lake to their needs and put the ecosystem under pressure, on the other hand these societies respond to the functioning of the lake ecosystem.
An analysis of the socio-ecological system of lakes should include insight into:
- the abiotic physical processes
- the biotic ecosystem processes
- the interrelations and feedbacks between the biotic and abiotic processes
- the governance structures and management systems (See tool for system analysis)
Ecology of lakes
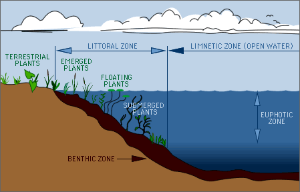
The biodiversity of lakes can differ greatly, not only between lakes but also within a single lake. Two key factors that determine the biodiversity in lakes are:
- The physical and chemical conditions within a lake and tolerances of different species to those conditions
- The abilities of different species to disperse into a lake.
Ecological zones
Generally four major ecological zones are identified in lakes (see figure on zones in standing water), providing different conditions for biodiversity:
- The littoral zone is the nearshore area where sunlight penetrates all the way to the sediment and allows aquatic plants (macrophytes) to grow. The littoral community is considered the most diverse and abundant biological community in lakes.
- The euphotic zone of the lake is the layer from the surface down to the depth at which light levels become too low for photosynthesis. Light intensity usually decreases exponentially with depth, due to absorption and attenuation by phytoplankton, suspended particles and dissolved organic matter. The limit for photosynthesis is the depth where around 1% of the incident light intensity at the water surface is still available.
- The limnetic zone is the open water area where light does not generally penetrate all the way to the bottom.
- The benthic zone is the surface layer of the bottom, with sediments abundant with organisms. This upper layer may be mixed by the activity of the benthic organisms that live there, often to a depth of 2-5 cm in rich organic sediments.
The zonation can be seen to be largely determined by the light extinction curve in the water column. If the light extinction coefficient increases, e.g. due to increased sediment resuspension or to massive algal blooms, the spatial delineation of the major ecological zones will also change: the littoral zone will shrink and the euphotic layer will narrow. Populations of macrophytes, phytoplankton and zooplankton will follow these changes in their distribution. Also fish and benthic life will be affected because the flux of organic matter (food) to the sediment will change.
For an overview of these zones see: www.lakeacces.org.
Lake food webs
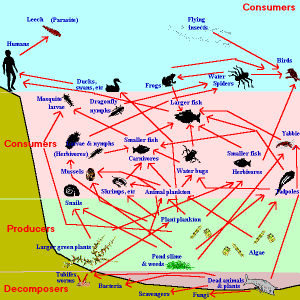
Aquatic plants and animals interact with each other through a series of interconnecting pathways called a food web. The various levels in the food web or chain are called trophic levels, each of which represents a different type of productivity. The interconnection between the trophic levels is often very complex and dependent on the local lake situation.
At the basis of the food web in the aquatic environment starts we find the primary producers: phytoplankton (predominantly algae) and macrophytes, that use sunlight, water and nutrients for photosynthesis. When either light or nutrients (or both) are in short supply, photosynthesis will be limited. In the littoral zone, where the water is shallow enough for macrophytes to occur, macrophytes and algae are in competition for light and nutrients. Primary production rates of macrophytes and algae, as well as the outcome of their competition, can be fundamentally changed by altering nutrient loading of the lake.
Phytoplankton is the primary food for many species of filter-feeding zooplankton (e.g. Cladocerans) and filter feeding benthos (e.g. Dreissena mussels). Zooplankton in turn is eaten by planktivores, that include fish as well as a variety of aquatic insect larvae. The piscivores, fish-eating fish, are at the top of the aquatic food web. Extending the food web to the terrestrial environment, it becomes even more complex, with a multitude of relationships with birds, reptiles, amphibians, mammals, spiders, insects and man.
Food webs of lakes can be fuelled by internal production, i.e. photosynthesis by plants and algae, but also by external production, i.e. carbon inputs from surrounding land.
Macrophytes
Macrophytes (large multicellular plants in lakes) play a very important role in the lake ecosystem. They add structure to the aquatic environment and support an abundance of life. In the littoral zone, many fish build nests in the vegetation and young fish find protection among the plants from predators. A multitude of aquatic insects (food for many fish) live on and feed among the plants. They also produce oxygen, which assists with overall lake functioning.
Macrophytes may be classified into several groups based on whether they are rooted (attached to substrate) or floating, and whether all parts remain submerged or some parts emerge out of the water (emergent).
The absence of macrophytes may indicate water quality problems as a result of excessive turbidity, herbicides or salinization. This may result in a reduced population of (sport and forage) fish and waterfowl (Crowder and Painter, 1991). An overabundance of macrophytes as a result of high nutrient levels may also interfere with the ecosystem services that a lake can provide, e.g. recreational activities (swimming, fishing, boating) and aesthetic appeal.
Microbial organisms
Microbial organisms present in most lakes include unicellular and colonial algae, rotifers, protozoa, bacteria and blue-green algae. Photosynthetic algae that float freely within the limnetic zone are referred to as phytoplankton. Dense blooms of phytoplankton may occur in lakes where nutrients are abundant, turning the lake turbid and green (eutrophic lakes).
Invertebrates
The invertebrate fauna of lakes mainly consists of crustaceans, molluscs, oligochaete worms and adults, larvae or nymphs of insects. The tiny animals suspended in the water column are called zooplankton . Like phytoplankton, these species have developed mechanisms that keep them from sinking to deeper waters, including drag-inducing body forms and the active flicking of appendages such as antennae or spines. Remaining in the water column may have its advantages in terms of feeding, but this zone’s lack of refuge leaves zooplankton vulnerable to predation.
The invertebrates that inhabit the benthic zone are numerically dominated by small species and are species-rich compared to the zooplankton of the open water. They include crustaceans (e.g. crabs, crayfish, and shrimp), molluscs (e.g. clams and snails) and numerous types of insects. These organisms are mostly found in the vegetation of the littoral and euphotic zone, where the richest resources, highly oxygenated water, and warmest portion of the ecosystem are found. The structurally diverse macrophyte beds are important sites for the accumulation of organic matter, and provide an ideal habitat. The sediments and plants also offer protection from predatory fish.
Vertebrates
A variety of vertebrate animals live in and around lakes. Species composition is very much dependent on the local situation.
Fish are a notable species group in lakes, and represent an important part of the ecosystem services that lakes deliver. Fish species have specific physiological tolerances and preferences in temperature, dissolved oxygen concentrations, and spawning needs. Because fish are highly mobile, they are able to deal with unsuitable abiotic factors in one zone by simply moving to another.
Other vertebrate taxa of lakes include amphibians (e.g. salamanders and frogs), reptiles (e.g. snakes, turtles, and alligators), and a large number of waterfowl species. Most of these vertebrates spend part of their time in terrestrial habitats and thus are not directly affected by abiotic factors in the lake or pond. However, food shortage in the aquatic environment can severely affect waterfowl, for instance, which are heavily dependent on the food source that delta waters normally provide.
Eutrophication
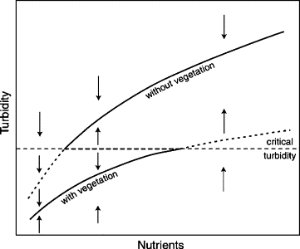
The effects of eutrophication (increased input of nutrients) into shallow lakes is reflected in the turbidity of the lake water as shown in the figure. When starting in conditions of low nutrient input and clear water (leftmost side of the lower line) macrophytes will dominate over phytoplankton as primary producers in the lake. With increasing eutrophication (following the line from left to right), turbidity will increase as phytoplankton production increases. When a critical turbidity threshold is passed (dashed horizontal line), macrophytes will disappear from the system and a switch will occur towards a lake without macrophyte vegetation and with high turbidity (upper line). Upon reduction of nutrient inputs, turbidity will slowly decrease until the critical turbidity level is passed again (following the upper line from right to left). At that moment the lake will switch states again and become dominated by macrophytes. This switch back from the turbid to the clear water state occurs at much lower nutrient input levels than the first shift from clear to turbid. That phenomenon (the ‘memory’ of the system) is called hysteresis.
Enrichment of lakes with nutrients can cause algal blooms. This pollution usually originates from agriculture, industry and urban centres. When nutrients accumulate, a lake ecosystem can quickly shift from an oligotrophic state (low nutrient levels, low productivity, clear water) or mesotrophic state (intermediate levels of nutrients, productivity and water clarity) to a eutrophic state (high nutrient levels, high primary production, low water clarity). Reduction of nutrient inflow (external loading) tends to be swiftly compensated by ‘internal loading’; the release of phosphorus stored in the bed sediments by wave and wind forces and foraging fish species. This is one of the reasons why bringing eutrophic and turbid lakes back to an oligotrophic or mesotrophic state is quite difficult and time-consuming. Ecological feedback mechanisms in lakes can be another reason why it is difficult to turn a eutrophic and turbid lake into an oligotrophic or mesotrophic and clear one.
Alternative stable states
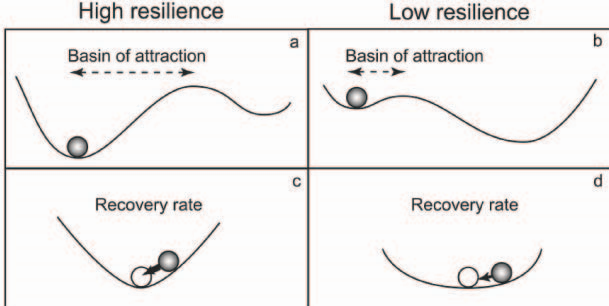
Shallow lakes can have two alternative equilibrium states: a vegetation-dominated clear state and a turbid non-vegetated state. These equilibrium states are correlated with the nutrient levels in the lake. However, when a lake is in one equilibrium state, it does not easily flip to the other (see figure on critical turbidity of shallow lakes). This resilience is the result of feedback loops in the ecosystem.
When nutrient levels increase, the density of algae increases and the water becomes more turbid. When a critical turbidity is exceeded, macrophytes will entirely disappear, as they do not receive light anymore (Scheffer et al., 2002). In turbid water, the number of piscivorous fish will decline, as these hunters need the submerged vegetation to hide in and need clear sight to see their prey. If the piscivore population is low, the number of planktivorous fish will increase, resulting in a depletion of their principal food source, the zooplankton population. Less zooplankton eat less algae, resulting in higher algae densities. Also benthivorous fish will profit, as they forage by touch and are not hindered by the turbidity of the water. When foraging they stir up the soil. This combined with the absence of macrophytes will increase the turbidity of the water also by floating sediment and keep the lake in a turbid state.
In a turbid lake, there is a relatively small bird community of piscivores and omnivores. Also, cyanobacteria often dominate the phytoplankton community if the lake is in a turbid state. In the clear vegetated state the fish community is more diverse, and large numbers of herbivorous and omnivorous waterfowl visit the lake (Scheffer et al. 2002). A turbid lake delivers far less ecosystem services than a clear lake can. See here for more information.
Physical drivers
The main physical processes in lakes are stratification, sedimentation and resuspension and wind-induced waves and currents.
Stratification
Thermal stratification is specific for deeper lakes. It is caused by temperature differences within the water body. As the water heats up during the warmer summer time, a temperature gradient (thermocline) may develop between warmer surface waters and cooler deep waters. This thermocline acts as a barrier to vertical movements within the water body. The top layer (epilimnion) may become nutrient-poor as aquatic organisms use all available supplies and cannot access those below. The bottom layer (the hypolimnion) may become depleted of oxygen (hypoxic) because of its isolation and may even become anoxic, producing hydrogen sulphide. The thermocline usually breaks down in winter or under strong winds, allowing vertical mixing: the movement of nutrients upwards and oxygen downwards triggers a period of renewed growth. A minimum depth is required for thermal stratification to build up. Shallow coastal lakes are often too shallow to show the phenomenon.
Sedimentation and resuspension
In many lakes, inorganic sediment particles, but also algal cells, go through a rapid cycle of sedimentation and resuspension. Resuspension is often caused by turbulence as a consequence of wave action but also fish searching for food in the bottom can stir up considerable amounts of sediments (see figure on turbulence). Whether or not wave action leads to resuspension of particles depends on the shear velocity and on the properties of the sediment.
Wind, waves and currents
Wind is generally the main cause of waves in lakes. The two main determinants of wave heights are the strength of the wind (speed and duration) and the length of the lake on which the wind acts; the fetch. The longer the fetch, the higher the waves. Waves generate a horizontal water movement along the sediment surface which causes the resuspension of sediment into the water. Wave action, also called stirring, causes erosion of the shoreline in lakes and reservoirs. The severity of erosion of the shores depends on the geometry and materials that make up the shoreline.
Other effects of wind acting on a lake are circulation currents and a water surface gradient. Wind sets down the water surface at the upwind side and sets it up at the downwind side. This can give rise to substantial water level differences and to a significant reduction of the wave-attenuating effect of wetlands, for instance. This determines the design conditions for lake-bordering flood defences.
As the wind force acts at the surface, it will drive a water circulation in the vertical plane. Moreover, the force is more effective in shallower water, which means that horizontal circulation currents will arise if the lake has deeper and shallower parts. Together with the wave-induced water motion, these currents contribute to the mixing of the lake.
Transitions
Many lakes once were lagoons with an open connection to the sea. If closed off artificially, lagoons will go into a transition and cause specific management problems and challenges. Specific problems are:
- A gradual decrease in salinity can create intermediate conditions that offer opportunities to noxious algae species, notably blue green algae. This may be a temporal state, but in some systems problematic conditions may prevail for decades. Management may focus on reinstating minimum salinity levels or increased flushing and reduced retention times.
- Clay sediments can lose their aggregate stability because internal chemical cohesion is reduced as salinity decreases. As a consequence the lake bottom sediment is especially prone to suspension. Over time an unconsolidated mud layer develops that, decades after the closure, suffocates all benthic communities in the lake, leading to the reduction in food sources for birds and a reduction in fish communities.
- Bottom sediments can accumulate phosphorus leading to enhanced internal P-loading that can be a major driving force for algae growth. The build-up of phosphorus can be gradual and water quality problems may become manifest only decades after closure. Problems can be severe to local fisheries, recreation and water supply. Management may opt for stringent emission control, increased flushing or even sediment management strategies. Also partially reopening of the closure dam is sometimes considered a management option.
- Loss of tidal activity often leads to the concentration of wave energy in a narrow zone resulting in erosion of former tidal flats and the need for costly shore protection measures.
- Loss of tidal activity may also lead to static and anoxic groundwater in riparian lands. Especially in lowland this process may affect large areas. Anoxic groundwater may lead to the die-off of root systems of trees and the geochemical release of toxic elements.
Governance processes
A characteristic of many lakes throughout the world is the fragmentation of management and administration. The governance of a lake and its coastal zones seldom falls within the competence of one organisation, and even in such cases management needs to handle competing claims and different perspectives on planning and decision making. Consequently every new initiative is target to a large number of organisations who want to influence planning and management decisions. Herewith, the decision making process becomes in a complicated interplay of parties whereby everyone and no-one feels responsible. In the face of fragmentation of management and administration, an integrated approach in planning and decision making is more appropriate in lakes than a sectoral one.
For more information on governance processes see Governance.
Ecosystem services
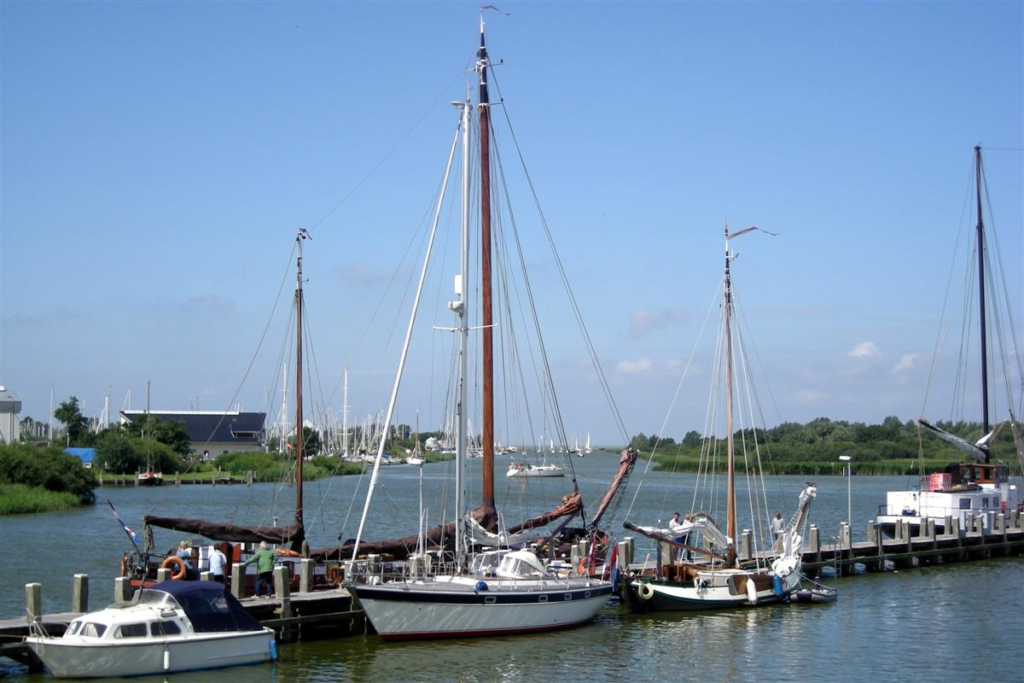
Ecosystems provide all sorts of benefits – like goods or services – for mankind; these benefits are known as ecosystem services. Generally these services are divided into four categories (United Nations Millennium Ecosystem assessment):
- Provision, such as the production of food and water
- Regulation, such as soil retention
- Cultural, such as recreational benefits and
- Support, such as nutrient cycles.
For further reading on ecosystem services see here (TEEB).
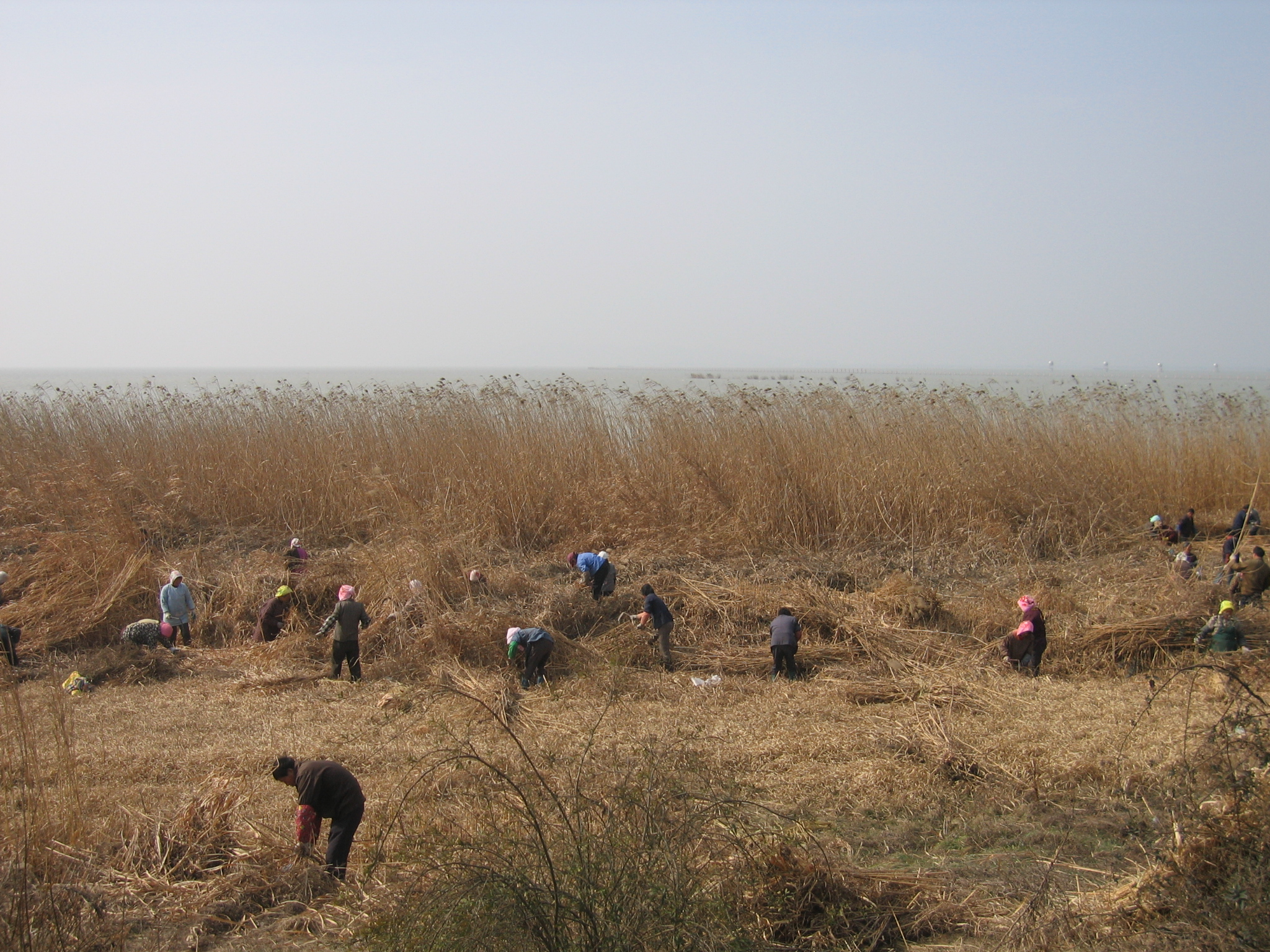
Provision
- Freshwater supply for domestic, agricultural and industrial use. The IJsselmeer, for instance, is one of the largest freshwater reservoirs in Europe, providing 30% of the Netherlands with irrigation water during dry summers, water to flush the land to prevent salinization of soils and drinking water for one million people.
- Fish production – fisheries are an important sector in many lakes
- Opportunities for tourism –this is also often an important economic sector.
- Supply of construction materials (mainly sand).
Regulation
- Protection of the hinterland against incoming storm surges by barriers and structures in lakes.
- Safety against flooding of inland areas by the storage capacity of lakes for river or precipitation water.
- Water storage of surplus water in winter or the rain season, to be used in summer or the dry season.
- Prevention of the discharge of sediment from the shores into the lake during flood events by the stabilizing effect of the natural riparian vegetation.
- Water purification and pollution control through absorption, filtering and dilution of nutrients, pollutants and other wastes.
- disease control by preventing the expansion of bacteria and microbes that possibly affect people.
Cultural
- Possibilities for recreational activities like sunbathing, swimming, fishing, bird watching, canoeing and yachting. A large part of the human population lives and works in cities near lakes, so this ecosystem service is of value for a lot of people.
- Spiritual inspiration attributed to a certain lake.
- A feeling of serenity and space.
- Landscape aesthetics.
- Cultural heritage and identity.
Support
- Nutrient cycling.
- Partly due to spatial differences in vegetation, lakes provide wide diversity of habitat to invertebrates and (juvenile) fish.
- Water cycling by storing, regulating and recharging surface and sub-surface waters.
- Many shallow foreshores offer nursery habitats for e.g. fish and oysters.
- Provision of nesting, resting and foraging habitat for waterfowl and migrating birds along dikes, dams, on berms/islands, flats.
- Habitat for freshwater species.
- Lakes may provide significant food supply to international populations of fish- and mussel eating water fowls.
- Natural, gentle slope of the shores result in large areas of shallow water that offers favorable conditions for aquatic and riparian plants to flourish.
Building with Nature opportunities
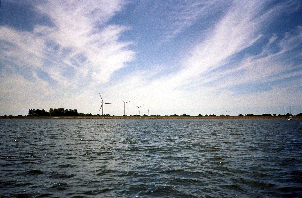
Worldwide efforts in lake restoration
Ecological restoration of shallow deltaic lakes is a topic of considerable interest. In particular the restoration of ecological quality after eutrophication events has attracted much attention (see Verdonschot et al., 2012, for a review of restoration efforts in different types of wetlands). In comparison, relatively few restoration efforts have aimed at improving the structural diversity of lake ecosystems. Recently, restoration of (riparian) wetlands with clear water purification and biodiversity functions has received considerable attention. In urban environments, lakes serve particularly valuable functions, including recreation, that have attracted a lot of attention in restoration efforts.
Restoring water quality in lakes is a pressing need in many places, as lakes serve important functions as sources of drinking water in urban areas. Eutrophication may lead to turbid waters with high phytoplankton content, which are expensive to filter and purify, but worse than that, harmful blooms of cyanobacteria may even lead to toxic waters and extreme shortage of drinking water. Water quality was the main reason for ecological lake restoration in the past decades.
External vs. internal measures
When lakes are eutrophied due to increased external nutrient loading, they may switch from a clear-water to a turbid state. This switch is not only related to the nutrient concentrations in the water received by the lake, but also to internal nutrient storage in sediments and biota. A prerequisite for lake restoration is the reduction of the external loading to a much lower value. As explained above (link to switching between alternative stable states) reduction to much lower input values than the ones present at the state transition from clear to turbid water is usually needed, due to hysteresis. However, it is often observed that reduction of external loading is not sufficient to reverse the state of a lake. The reason is that the internal nutrient accumulation continues to provide a rich nutrient source for very long times. In particular, phosphorus stored in sediments is recycled during the growing season and can maintain a high production of phytoplankton.
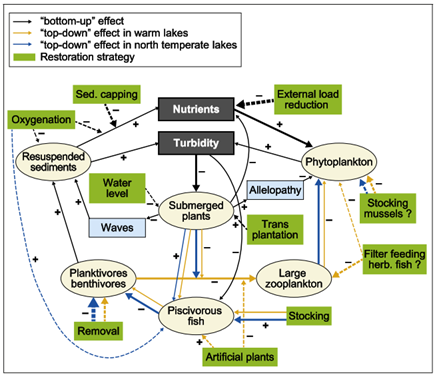
For this reason, lake restoration often combines external measures (reduction of nutrient loading) with internal measures, referred to as biomanipulation. Different biomanipulation methods have been described in an extensive literature. Jeppesen et al. (2012) provide a comprehensive review of biomanipulation methods and their relative success rate in many example cases. An overview for temperate and warm lakes is provided in the figure.
Overview of biomanipulation methods in temperate and warm lakes, with an indication of the major feedback loops affecting the state of the lake. The strength of the connections is indicated by the width of the lines. Colour coding of the lines indicates differences between temperate and warm lakes. Figure is taken from Jeppesen et al. (2012) and is based on Scheffer et al. (1993) as modified by Meerhoff and Jeppesen (2009)
Manipulation of fish stocks is the oldest and most often applied method of biomanipulation. Planktivorous and benthivorous fish are removed from the system, while piscivorous fish are favored by improvement of their habitat or by additional stocking. As a consequence, predation on zooplankton is reduced, and this zooplankton exerts stronger control on the phytoplankton. This increases transparency of the water and favors development of submerged macrophytes. These in turn have a positive effect on water transparency by reducing wave impact on the sediment. They also provide structural habitat for piscivorous fish.
Additional biomanipulation measures can be added to this scheme. Stocking with zebra mussels can introduce additional grazing on phytoplankton and thereby reduce its effect on water transparency. However, it also results in fast recycling of nutrients in the system and can therefore not avoid sustained high primary production by phytoplankton or phytobenthos. In warm lakes, introduction of herbivorous fish can have a similar effect. In addition, many of these species are omnivorous and also feed on zooplankton. Macrophytes can be introduced into the system, which is particularly effective in places where propagules are lacking.
The schemes can further be completed with physical and chemical interventions. Manipulation of water level may change the growing conditions for macrophytes and favor their development. Oxygenation of bottom waters, especially in deeper lakes with anoxic conditions near the sediment, has large influence on the regeneration of phosphorus from the sediments, as well as on the survival of piscivorous fish. However, it is a very expensive and energy-intensive intervention that has to be maintained for prolonged periods. Sediment capping, in particular with substances that stably bind phosphorus, e.g. calcite (CaCO3), hydroxides of oxidised iron (Fe3+) and aluminium (Al3+) can be effective for several years, but usually has to be repeated over the years. The methods are most effective when a full state transition occurs and development of macrophytes stabilizes and oxidizes sediments.
Due to the many internal relations and the fact that biomanipulation interventions often have to be repeated over the years, the time scale of lake restoration is long, in the order of decades. On this long time scale, the effectiveness of the external measures (reduction of nutrient loading to the lake) are usually the dominant factor, and persistent success depends on the effectivity of these measures.
The potential role of connected wetlands
In recent years, a lot of attention has been devoted to Nature-Based Solutions (Building with Nature approaches) to the reduction of external nutrient and sediment loading to lake ecosystems. Whereas for important point sources of nutrients (urban agglomerations, industrial plants) technical measures for waste water treatment are usually most efficient, nature-based solutions may be very effective for diffuse sources, such as fertilizer leaching or soil erosion in agricultural fields. In particular, shallow marshy wetlands have been used to remove or withhold high percentages of nutrients and prevent their throughflow to lakes.
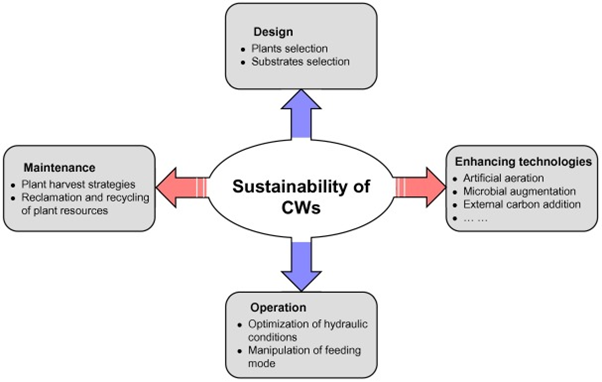
Summary of the challenges and future research needs in order to improve the sustainability of constructed wetlands for water purification. From Wu et al., 2015.An extensive treatment of wetland ecology, including design and use guides for constructed wetlands aiming at wastewater treatment, is given by the American Environmental Protection Agency (https://www.epa.gov/wetlands). Wu et al. (2015) give a recent review of the design, operation and technical developments of constructed wetlands for water purification. The figure below is drawn from this publication and summarizes the current challenges to be addressed in order to improve the long-term efficiency of these systems for their purpose.
Restored natural wetlands, as well as constructed wetlands, not only offer possibilities for water purification at a relatively moderate cost. They can also substantially contribute to the biodiversity of a landscape, by providing unique habitats of great value. This is especially the case in semi-arid environments. However, the design of wetland restoration has to be executed with great care, taking into account the watershed relations, the natural background numerous design principles as well as support from local communities. We refer to the sites of EPA on wetlands for extensive documentation of these principles. Extensive guidelines and principles for wetland restoration are also provided by the RAMSAR convention on protection of international bird populations (link). A conceptual review, including reference to the required ecological theory for successful restoration, is provided by Zedler (2000).
Urban lakes and wetlands
In recent years, particular emphasis has been placed on the restoration of rivers and lakes in urban environments. In addition to improvements of water quality and biodiversity, these restoration projects can also deliver crucial contributions to flood control and protection in a largely built environment, climate change adaptation by provision of retention areas, and leisure and recreation services for the dense population of the areas. That makes restoration of urban lakes a particularly beneficial activity. Urban lake restoration typically involves changes to the physical structure of the lake, in particular of the riparian zone, as many urban lakes have previously be banked with highly artificial (often concrete) shores. The attractiveness for recreation, as well as the biodiversity values, can greatly be enhanced by reconstructing shallow marshy shores. The water storage capacity in urban environments is also of great importance, in particular since more intense precipitation bursts are expected with climate change. Storage of storm waters in marshy ponds that can provide pretreatment of these waters before releasing them into the lake, is of particular importance.
An extensive description of guidelines and illustration with real-life examples is provided by the European Environment Agency in a recent report (EEA 2015).
EcoShape Building with Nature examples
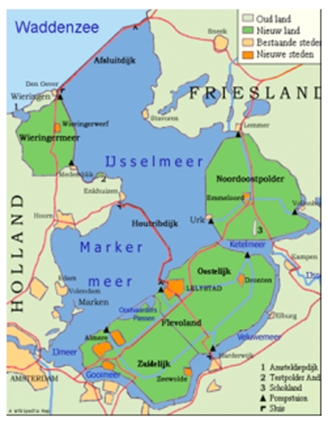
EcoShape experience of Building with Nature efforts in lakes is largely restricted to the artificially constructed lakes IJsselmeer and Markermeer in The Netherlands. In addition to water quality problems, these lakes suffer from transitional problems (link), lack of structural heterogeneity, and lack of connectivity. Maintenance and upgrading of dikes and flood defences offer opportunities for win-win solutions using the Building with Nature approach.Below some approaches to lake restoration using Building with Nature will be illustrated, using IJsselmeer and Markermeer as examples. The opportunities will be discussed in four themes: water quality restoration, soft flood defences, creation of spatial ecological structure and restoration of sea-river connectivity.
Water quality restoration
Approaches to ecological restoration after severe eutrophication leading to a system switch towards the turbid stage (link to alternative stable states here, see above) have followed different paths. Hilt et al. (2018) extensively discuss the pros and cons of these approaches. They distinguish external and internal measures. External measures consist of strong reductions of the nutrient loading of the system, by water purification or flushing of the lake with nutrient-poor water. Internal measures often concern biomanipulation, where fish populations are thinned so as to reduce the predation pressure on zooplankton by planktivorous fish, and the intensity of bottom sediment reworking by benthivorous fish. Other lake-internal measures consist of fixing the phosphorus in the sediment, e.g. by binding it to Al complexes or other stable chemicals, or removing top sediment layers with their high internal phosphorus concentrations.
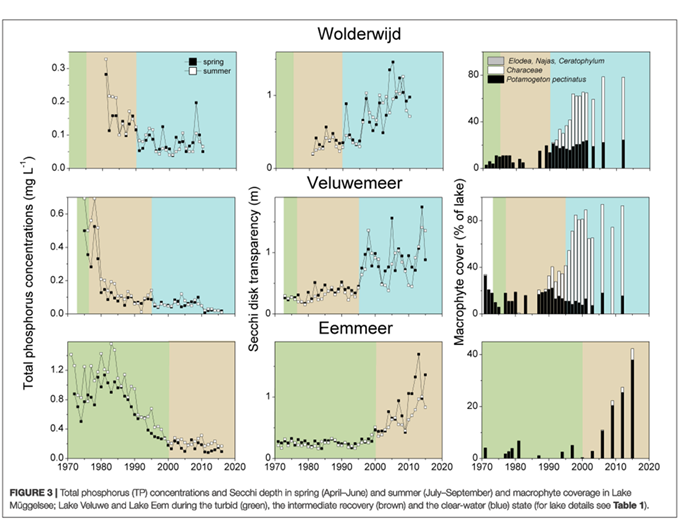
Hilt et al. (2018) conclude from an extensive model-supported meta-analysis that external measures often lead to an intermediately disturbed state with clear water in spring but continuing turbid water in summer, as they tend to promote macrophytes with a predominant spring development, while in summer nutrient loading from internal sources continues to favor phytoplankton dominance. Treatment with lake-internal measures only tends to lead to a system switch towards macrophyte dominance in the short term, but reversion to the turbid state after a number of years is often observed. Combination of both measures, i.e. internal measures that prevent the continuation of phytoplankton dominance and the mobilization of internal nutrients, while ensuring structurally lower nutrient input by external measures for the longer term, turn out to be the most successful approach.
The switch from turbid to a clear-water, macrophyte-dominated state is illustrated for three marginal lakes of lake IJsselmeer in the figure below. Of the three lakes, Wolderwijd and Veluwemeer were actively flushed with nutrient-poor water during the period 1982-1989. In addition, Wolderwijd received biomanipulation in 1990. Eemmeer was only treated by reducing external nutrient inputs. Its present state is still in ‘intermediate recovery’ modus, even though there are moderate signs of further improvement, e.g. by the observation of the first Characeae around 2010.
We refer to Hilt et al. 2018 and the references in that paper for detailed discussion of these and other examples.
Soft flood defenses
The littoral zone has special value and functions within shallow lakes, due to its role as habitat for fish reproduction and its functions for stabilization of suspended sediments and dominance of structurally rich macrophyte zones. As discussed earlier, the spatial extent of the littoral zone is a function of the steepness of the bathymetry and of the water turbidity, as it is defined as the zone where the sediment surface is within the euphotic layer. In turbid lakes with steep side walls, the littoral zone is very narrow and very little opportunity for the development of a stable macrophyte-dominated littoral zone exists. The steepness of the bathymetry does not only limit the extent of the littoral zone, it also concentrates the dissipation of wave energy into a very narrow band, thus leading to erosion of the lake shore and further increase of the steepness of this zone.
In turbid lake Markermeer, these features of a very steep and ecologically poor littoral zone are most expressed along the eastern border, which is made up of an artificial dike built in relatively deep water. For reasons of flood defence, this dike was in need of maintenance and strengthening. Based on the Building with Nature approach, a soft solution consisting of the deposition of a sand body in front of the dike has been preferred over a traditional dike reinforcement.
The following diagrams illustrate two of the intended measures. A sandy littoral zone with a very mild slope will be constructed in front of the dike at both sides, wherever the depth of the water allows for this solution to be physically stable. Only where water is too deep and around sluices, stones will be used as the building material.
In addition to the reinforcement of the dike along its entire length, an extended littoral zone will be constructed at one pilot location. This extended littoral zone will consist of a number of low islands with very shallow water in between, favourable for the development of reed marshes. It will enrich the biodiversity of the lake, as well as constitute a sand reserve for the expected sand transport in the along-dike direction. More information is given here.
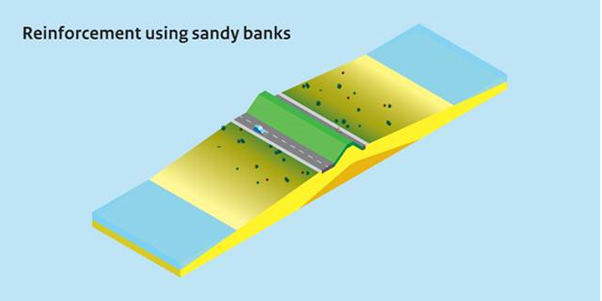
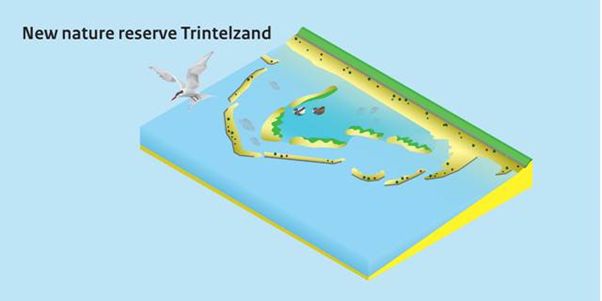
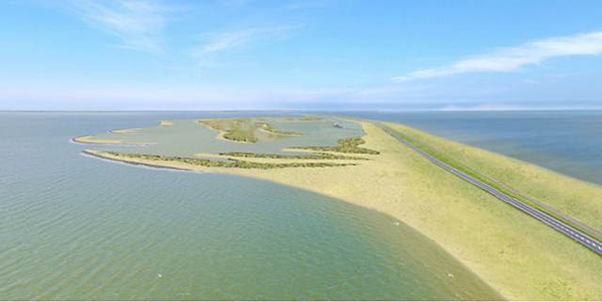
Along the eastern shore of the IJsselmeer two additional pilot projects were executed: the construction of Sand Motors to protect the coastline. They consisted of sand nourishments in front of the shore (Workumerwaard) or touching the shore (Oudemirdum) and served the double purpose of providing additional sand to the shoreline to compensate for erosion, and creating shallow-water zones for the development of macrophyte-dominated littoral communities.
The experiences with the nourishment were not very positive. They were subject to wave erosion, but the sand was not transported towards the shoreline in any significant amount. Also the creation of protected zones for ecological development was relatively unsuccessful. There are no concrete plans for the continuation of this approach.
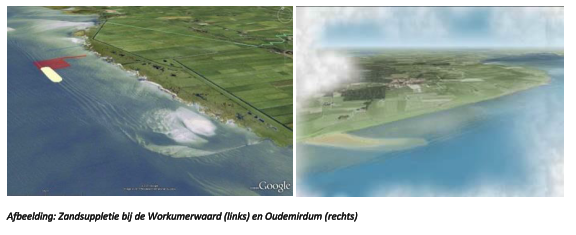
Creation of spatially-structured habitat complexity: Markerwadden
The uniform, shallow bathymetry of lake Markermeer, combined with the weakening of former marine clays in a freshwater environment (link) and the lack of stable accommodation space for the deposition of suspended sediments, is a factor strongly enhancing the turbid nature of the water, in addition to eutrophication phenomena. A large-scale (surface) restoration project aims at improving these conditions, as well as offering new breeding, resting and foraging space for internationally protected bird populations. In the ‘MarkerWadden’ project, a series of artificial islands and shallow submerged areas are constructed. The construction process uses sand for the outer linings of the islands, whereas soft mud dredged from the lake bottom is used as infilling material. This construction method is experimental, as is the approach to ecological restoration.
The sand mining for the island has created a deeper pit in the lake, that is intended to function as a mud trap to be regularly dredged as a source of additional filling material when the islands consolidate. In this way it is hoped that a substantial fraction of the soft, fluffy muddy material impacting the water turbidity will be removed. In addition, the shallow submerged parts between the islands will favour wetland development and further trapping of mud. The higher islands will serve as bird sanctuaries in the first place. Littoral zones around the islands should favour the development and reproduction of fish.
At present (2018) the construction of the first part of the plan is ongoing. Based on experiences gained in this first phase, the further plan will be adjusted and executed in later stages. The sand ridges have already attracted a large population of breeding birds, few months after their creation. More information ca be found here.
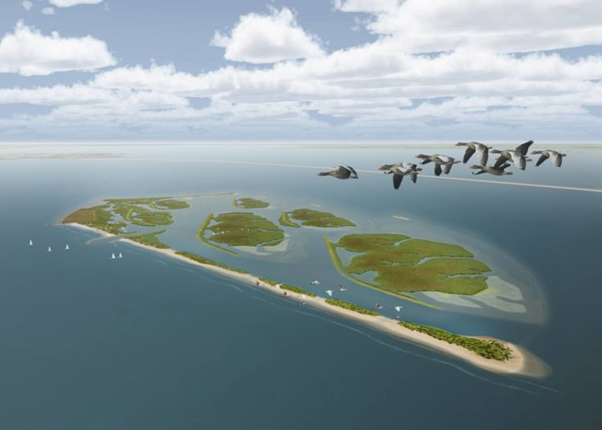
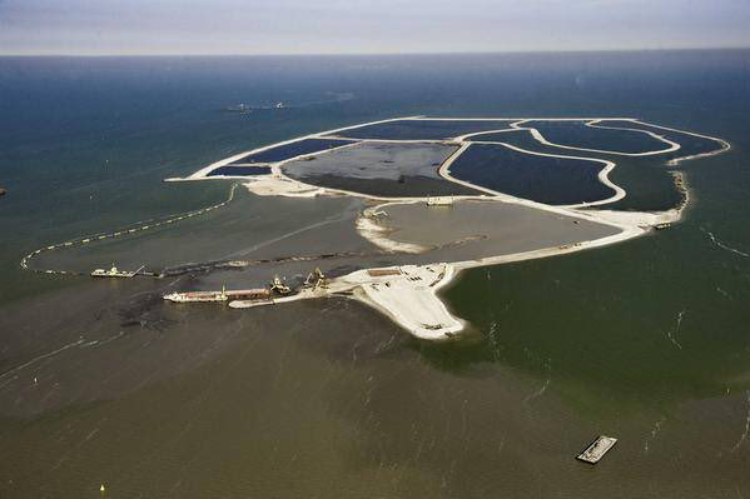
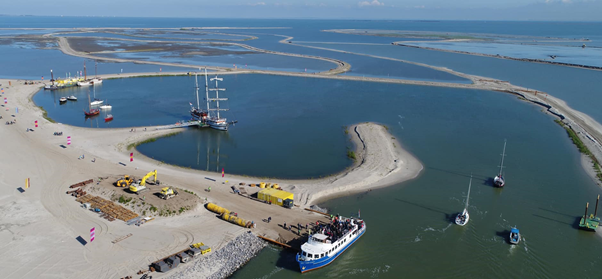
Restoration of sea-river continuum: the Fish Migration River
The Afsluitdijk, separating lake IJsselmeer from the Wadden Sea, constitutes a strong barrier for fish migration and is one of the factors inhibiting the restoration of salmonid populations in the Rhine catchment. In order to restore connectivity, plans have been made (and will shortly be executed) to restore migration routes for fish. The construction of a tidal fish migration passage is a much bigger challenge than conventional freshwater passages. The so-called ‘Fish Migration River’ is a long, winded ‘river’ with a narrow opening into the lake on one side, and into the Wadden Sea on the other side. There will be a continuous discharge of freshwater through the ‘river’, while the seaward end will be subjected to the tide. Within the ‘river’, salt and freshwater will mix, making it a miniature folded version of an estuary. It is calculated that there will be a sea-lake connection during most of the time, without risk of transport of salt water into the lake. More information can be found here.
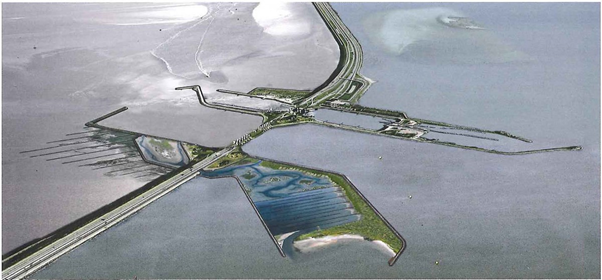
Lessons-learned
The lessons learned are specifically derived from the pilots and historic cases under the Markermeer-IJsselmeer case, and refer to the physical, ecological and governance processes.
Physical processes
- Sand nourishments at locations with a low level of hydro-dynamics can create new shallow zones and habitats by dispersion of the sand.
- Sand nourishments at locations with a more dynamic water motion may completely vanish from the shore.
- Local system behaviour is subordinate to larger-scale system processes such as currents, water level set-up and pollution. Interactions between higher and lower level system behaviour need to be understood in order to enable sensible planning and design.
- Forecasting of sediment transport and morphological processes in low-dynamic areas is complex. Specific hydro-morphological knowledge and tools are needed to understand them. Monitoring and data analysis are therefore important whenever an experiment is carried out.
- Wind offers additional dynamics to the exposed shallow shores along the coast. It may change the soil profile and the balance between erosion and accretion of sand. These dynamics create favourable conditions for a high-value nature development.
- Wind lowers the water level at the upwind side of the lake and sets the water level up at the downwind side. This may yield substantial water level differences in the lake and result in a significant reduction of the wave-attenuating effect of wetlands on the downwind side. These wind effects should be taken into account in the design requirements for lake-bordering flood defences.
Ecological processes
- Shore nourishments are maintenance measures. Initially they contribute to creating new terrestrial habitat with pioneer vegetation. In the longer run, they tend to spread alongshore and over the shoreface, thus contributing to sedimentation in the coastal zone on local and regional scales and offering possibilities for water-, marsh- and shoreline vegetation to develop.
- A slow decrease in salinity can create favorable conditions for toxic algae species, notably blue green algae. This may be temporary, but in some situations these conditions may prevail for decades. Management options are to set minimum salinity levels or to increase the flushing velocity, thus reducing the retention time of the water.
- As salinity decreases, clay particles can lose their cohesion as a result of a chemical process. As a consequence, the lake bottom sediment is prone to suspension and over time an unconsolidated mud layer may develop at the bottom of the lake. This mud suffocates benthic communities, leading to the reduction in food availability for many species.
- Bottom sediments can accumulate phosphorus elements. This may cause an enhanced internal P-load that can be a major driving force for algae growth in the lake. The build-up of phosphorus in the sediment is gradual and can cause water quality problems even decades after the separation of the lake from the sea. The decrease in water quality can cause severe problems for fisheries, recreation and water supply. Management options are a stringent control of emission, increased flushing or even sediment management. Also inlet of seawater by partially opening dams is sometimes considered an effective option.
Governance processes
- In the face of fragmentation of management and administration, an integrated approach in planning and decision making appeared to be more successful in lakes than a sectoral one, because:
- urgent short term objectives in spatial planning for certain land use functions can be connected with longer-term strategies e.g. for safety against flooding and fresh water supply;
- knowledge of a diverse group of stakeholders (e.g. local lake users, research scientists, community members with traditional knowledge, government representatives) becomes available in the project development process, which leads to more and better underpinned options and better supported decisions;
- it can lead to multifunctional projects and win-win situations. For example, the construction of shallow foreshores in the IIsselmeer (the Netherlands) was as a safety measure that contributed to the ecosystem functioning of the lake, increased the spatial quality of the area and promoted the multifunctional usage of the area (Groot et al., 2012).
- Well-considered framing of the tasks and a clear project mission is of crucial importance. A scope defined too narrow misses out potential opportunities. Therefore, integration needs to be sought in the process of decision making in order to find win-win conditions.
- Be clear about the objectives and find commitment of stakeholders on these. For instance, in the IJsselmeer area, the fact that all parties concerned expressed the objective to create conditions for fish migration gave the project an impulse.
- Pay attention to the consultation of authorities from the beginning of the planning and design phase. Timely start and manage formal procedures. In many stakeholder settings, authorisation requests and permit procedures are rigid and time consuming, with unexpected turns and developments.
- Start in the initiation phase with a small creative project team with a wide range of expertise and a mandate to make its own decisions. This enables them to quickly respond to unexpected developments.
- Multi-stakeholder involvement leads to a longer planning and implementation period, but it usually saves time during permit acquisition procedures and execution of works.
- Carefully consider stakeholders’ interests and translate those interests in multi-functional designs.
- Decision making, legal procedures and networking are part of the BwN design and implementation process. When dealing with conflicting interests three purposefully implemented strategies proved helpful:
- Beware of closing design discussion too early by presenting too unambiguous pictures, clear definitions, representations and technical designs. By keeping options open and somewhat vague, potential partners have the opportunity to translate their own interests into the plans, thereby avoiding early clashes on end results.
- Produce a short movie with decision makers expressing the importance of a BwN approach to the project. It shows the support of high-ranking officials for the experiments provides informal legitimation and facilitates negotiations.
- Arrange political and high level sponsorship by inviting officials to visit the project area.
- Communicate at multiple levels. Involve authorities at regional and sub-local levels as well as local stakeholders. Especially local stakeholders tend to be overlooked in (often complex) discussions and decision making processes at higher scale levels. Create a ‘Community of practice ’.
- A joint problem or opportunity, such as the BwN experiments, stimulates cooperation between stakeholders. A covenant in which rules of the game are made explicit, including funding responsibilities, favour an effective cooperation between stakeholders. Consider options for ‘Financing’.