Rivers are the conveyor belts that collect and transport excess precipitation and denudation products from the continents to the oceans. By transporting water and sediment, they create their own shapes through processes of erosion and sedimentation. Rivers transport also nutrients and seeds. Moreover, they sustain the lifecycles of fish by providing the routes for migration.
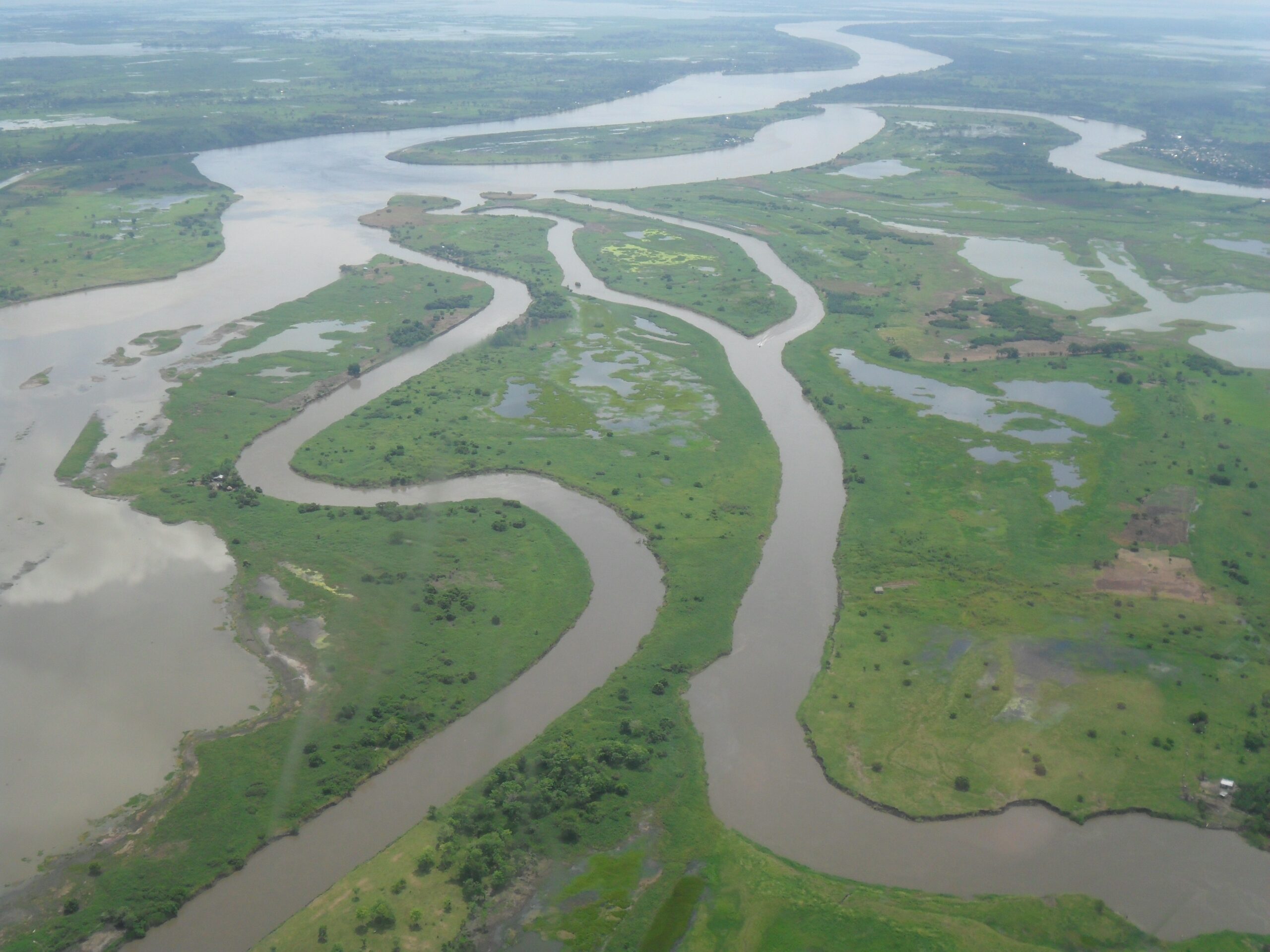
Rivers display a great variety in appearance. Their beds can consist of boulders, cobbles, gravel, sand or peat. They can be sinuous or straight. They can consist of a single, often meandering, channel or multiple channels. Multiple channels around bars that emerge above the water level at low flows characterize braided rivers. Multiple channels around more permanent and usually vegetated islands characterize anabranched or anastomosing rivers (Makaske, 2001).
Temporal and spatial differences in water depth, flow velocities and inundation frequencies produce variations in habitat along the river as well as across the river. The corresponding dynamics and gradients sustain rich and diverse ecosystems with a high intrinsic value. Moving from headwaters to the river mouth, water discharge gradually increases and sediment characteristics gradually change. Communities of flora and fauna change gradually too along with these changes in the physical environment, with gradual changes in the proportion of the four major food types: shredders, collectors, grazers (scrapers) and predators (Vannote et al, 1980). In lower reaches, tidal rivers form the transition from fluvial environments to estuarine environments.
Seen as the cradle of civilization, rivers have been providing ecosystem services to mankind for millennia. These services include freshwater supply, fish, transport, construction aggregates and hydropower. Additionally, rivers have profound historical, spiritual, artistic and aesthetic values.
System description
Rivers constitute a socio-ecological system driven by
- abiotic physical processes
- biotic ecosystem processes
- interactions between the biotic and abiotic processes
- governance structures and management systems
The combination of these aspects create a highly complex and dynamic environment, where human interventions can have an impact on a number of these system components on a range of temporal and spatial scales.
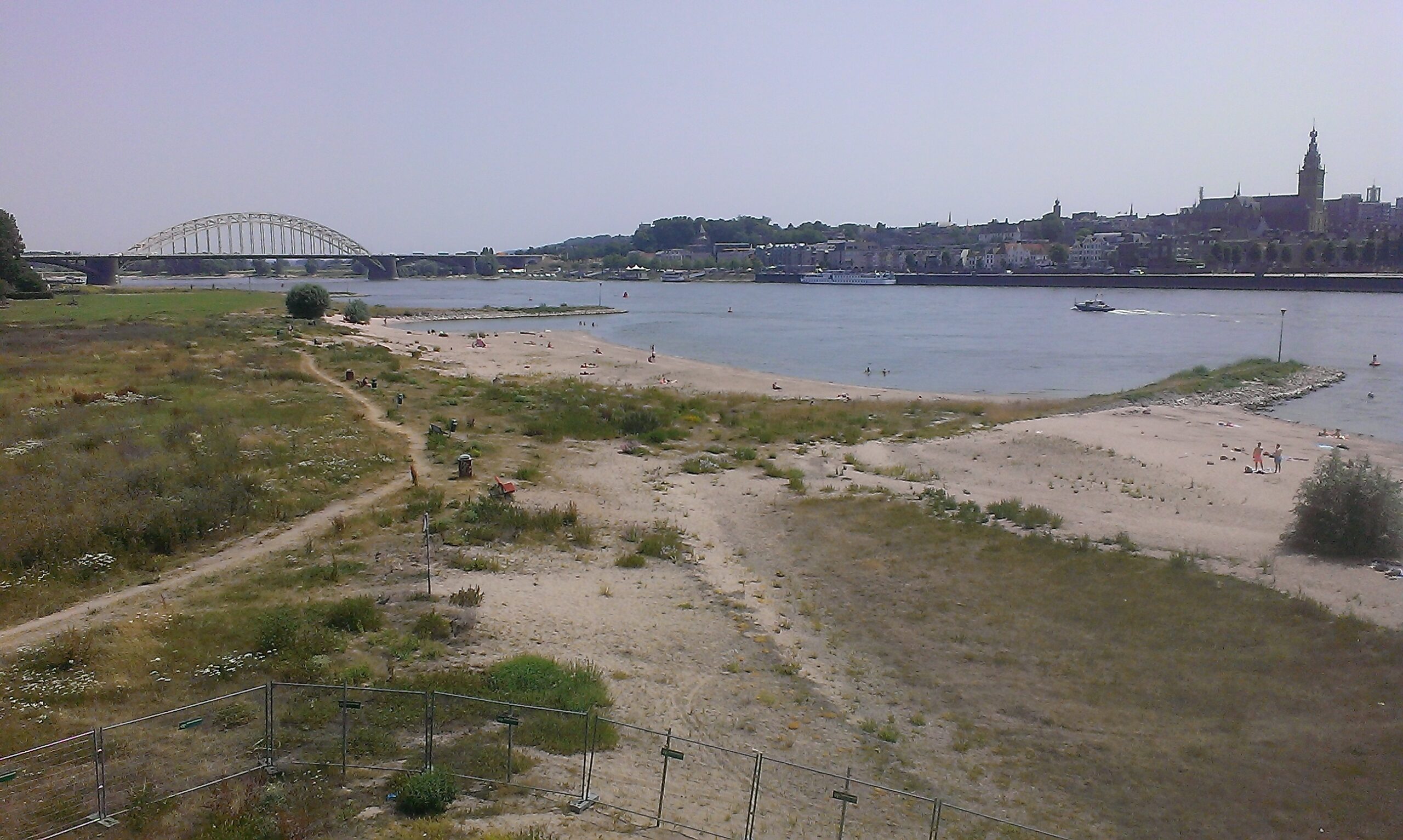
Physical processes
The main physical drivers of rivers are the discharge of water and the transport of sediment. The flow of water is driven by gravity and counteracted by resistance due to grain texture, bedforms, obstacles and vegetation. Sediment is transported by tractive forces exerted on the alluvial river bed or by forces on grains kept in suspension by upward motions in turbulent flow. The transport of coarser sediment depends on flow strength, leading to erosion of the bed in accelerating flows and sediment deposition in decelerating flows. Variations in flow velocity thus drive morphological changes that, on their turn, affect the variations in flow velocity. These close interactions regard the coarser bed-material load that can roll, slide and jump over the bed (bedload) or move in suspension (suspended load). Finer washload is transported in suspension too, but this transport does not depend on flow strength but on the supply of material upstream. Washload travels unaffected by variations in flow strength through the river and settles only in stagnant water bodies of wetlands or floodplains after floods. Fine cohesive sediments can also settle when they flocculate in the saline environment of estuaries. Mixtures of sediments with different grain sizes or different mass densities can segregate and give rise to spatial sorting patterns.
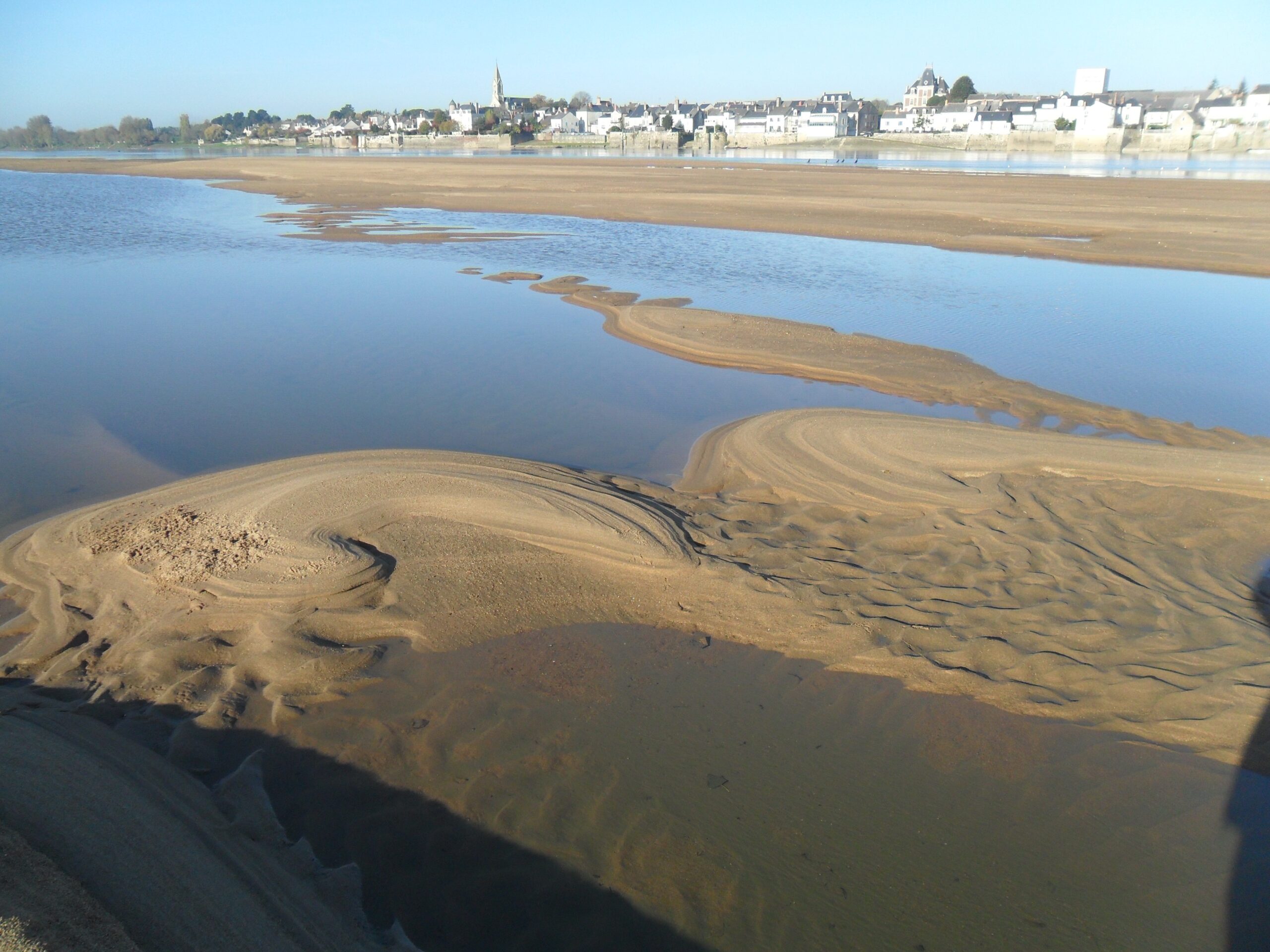
Morphological processes form shapes on different scales from ripples and dunes on the river bed, through bars and channels, to different planforms (Wright & Crosato, 2011). River planforms can be straight or sinuous (meandering), and they can be single-thread or multi-thread (braided, anabranched, anastomosing).
The morphology of rivers develops by interplay of water, sediment and riparian vegetation (Van Oorschot, 2017). Natural changes or human interventions can affect the interplay and thereby trigger a morphological response of changing bed topography, changing planform, changing bed sediment composition, or changing vegetation cover. Changes and interventions can occur locally, but also far away upstream or downstream. The time to develop a morphological response becomes longer as the distance from a change or intervention increases, carrying the risk that long-term river responses are overlooked in planning, implementation and post-project monitoring. Natural changes triggering morphological response include climate change, sea level rise, delta formation and subsidence. Human interventions triggering morphological response include dams, river training, flood embankments, water abstraction, dredging, sediment mining and encroachment into floodplains, as well as the anthropogenic components in climate change and sea level rise. The interventions and the associated morphological response put pressures on the fluvial ecosystem.
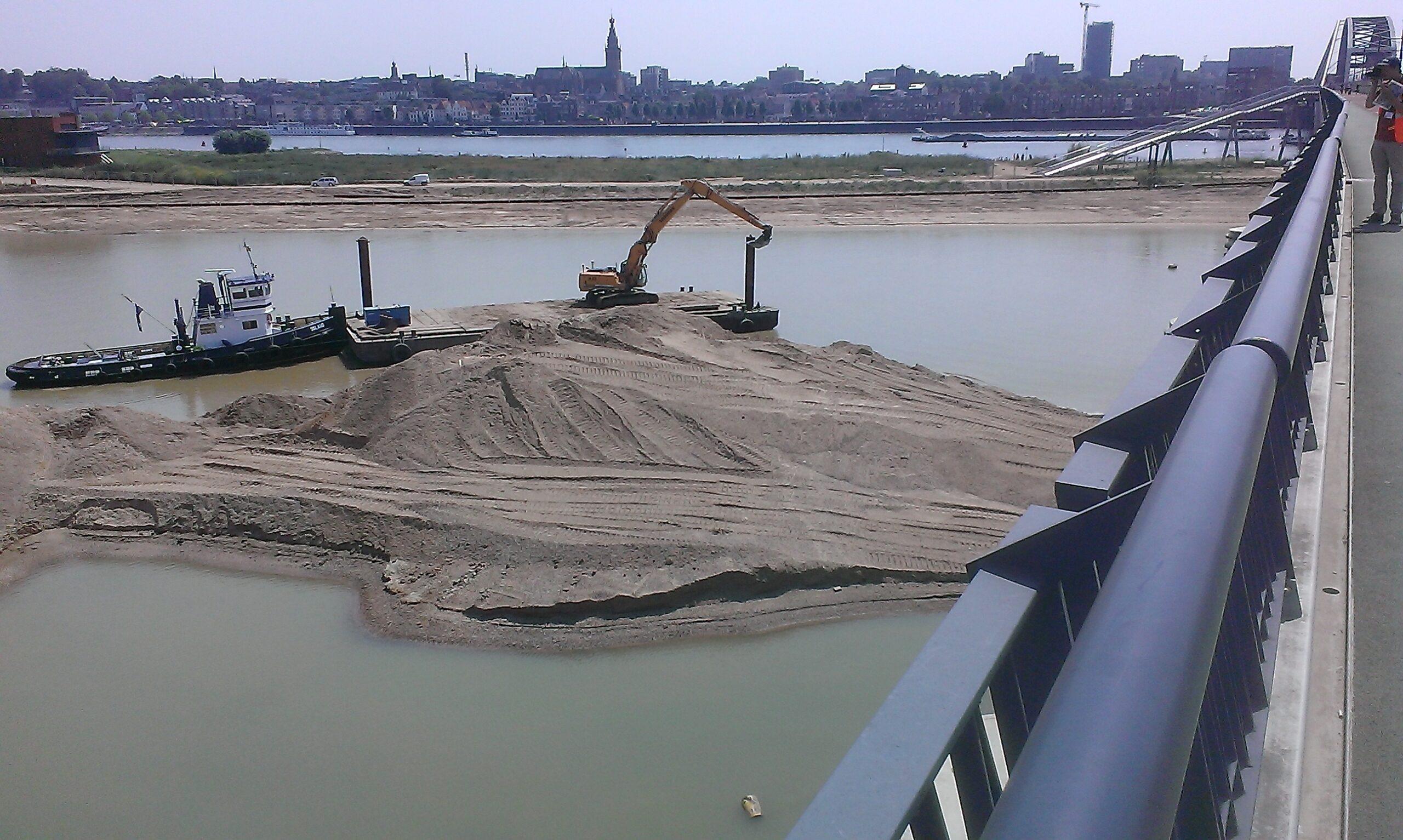
Ecosystem processes
The ecological functioning of rivers can be understood from specific fluvial ecological concepts in addition to general notions of habitat diversity and environmental stress:
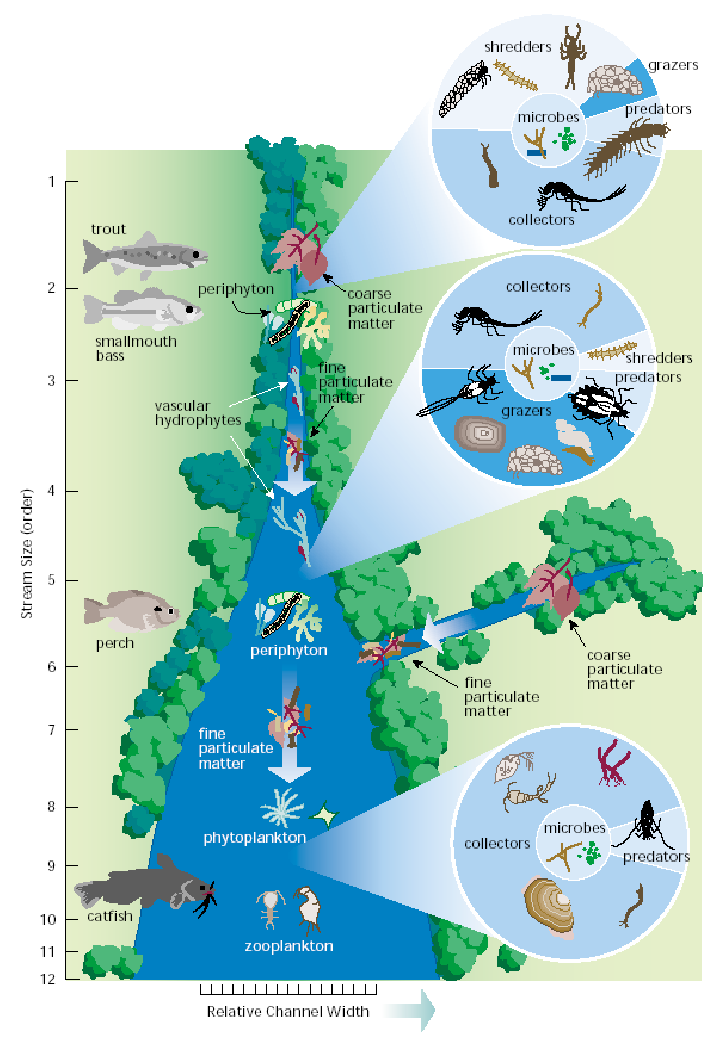
- The river continuum concept is a model for classifying and describing rivers on the basis of the occurrence of indicator organisms (Vannote et al, 1980). It assumes that structural and functional characteristics of stream communities are linked to physical parameters such as river width, depth, flow velocity and sediment load. These characteristics change gradually along the river as the physical environmental conditions change gradually from the headwaters to the lower reaches, also changing gradually the relation between production and consumption (respiration).
- The flood pulse concept is a theory that the annual flood pulse is the most important factor in driving and sustaining a fluvial ecosystem (Junk et al, 1989). It describes the movement, distribution and quality of water in river ecosystems and the dynamic interaction in the transition zone between water and land. Flood pulses cause periodic alternations of inundation and drying (Junk et al, 1989) as well as cycles of erosion and sedimentation (Tockner et al, 2000).
- The serial discontinuity concept is a reaction to the idealized picture of the river continuum concept (Ward & Stanford, 1983, 1995). It recognizes that rivers come in all shapes and sizes and that one size does not fit all. In particular, it considers the effects of weirs and dams that disconnect sections of the river upstream and downstream and displace resource gradients.
Connectivity is not only relevant in streamwise direction along the river. In transverse direction floodplains may be disconnected from the main channel by incision of the main channel or the implementation of steep hard bank protection. Different rivers may have different species of flora and fauna and yet have very similar ecosystems in terms of interrelationships and functioning. This can be addressed properly by studying guilds and traits rather than specific species.
Interactions between biotic and abiotic processes
River morphology evolves as a result of interactions between water, sediment and riparian vegetation (Van Oorschot, 2017). For the same hydrological regime and sediment loads, free vegetation growth results in a single-thread meandering river whereas the absence of vegetation produces a braided river (Tal et al, 2004; Tal & Paola, 2007; Crosato & Samir Saleh, 2011). Vegetation can enhance the morphological response to interventions by positive feedback. For instance, river incision due to bed erosion exposes shallow bars more permanently. The vegetation colonizing these bars concentrate flows even more, enhancing further bed erosion (Van Oorschot, 2017).
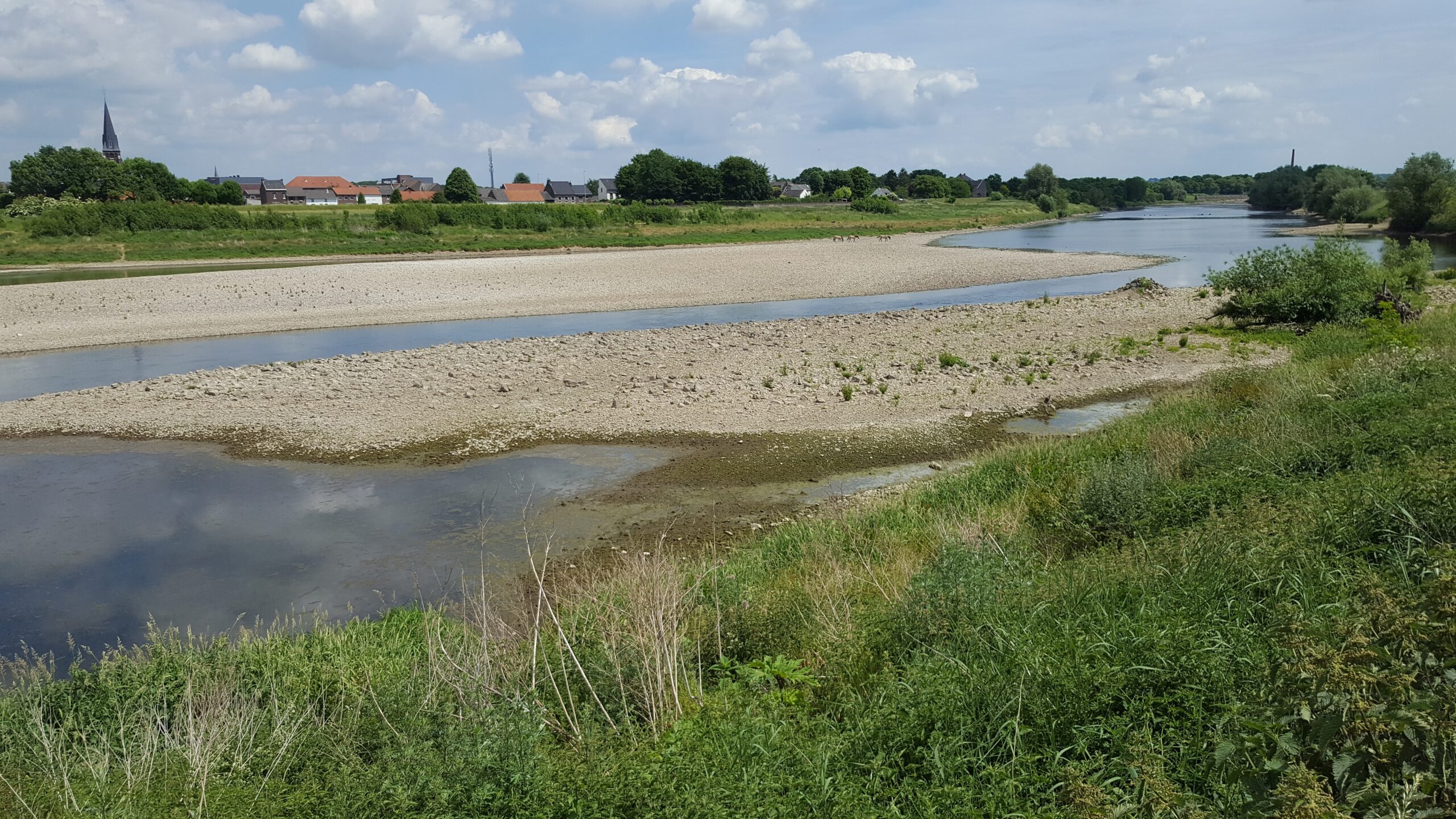
Governance and management
As rivers have been providing so many different ecosystem services since the dawn of civilization, it will come as no surprise that a multitude of actors and stakeholders plays a role, posing great challenges to governance and management. Here the corresponding governance structures and management systems are not discussed in detail. An illustration of their particular importance for rivers may be that, when the Netherlands was still a decentralized union of autonomous provinces, it was a river management conflict between the provinces of Holland and Gelre that, in 1798, gave rise to the installation of the first central governmental organization in the Netherlands. This “Bureau voor den Waterstaat” had to find a compromise between the interests of the two provinces. It was renamed “Rijkswaterstaat” in 1848 (Van de Ven, 1976).
Ecosystem services
Rivers have been providing a wide variety of benefits to mankind for millennia, making rivers the cradle of civilization. Rivers have always been an important source of water as well as an essential transport route for humans as well as aquatic life. For mankind, rivers also have great recreational and cultural values. These benefits, and others, are known as ecosystem services, commonly divided into:
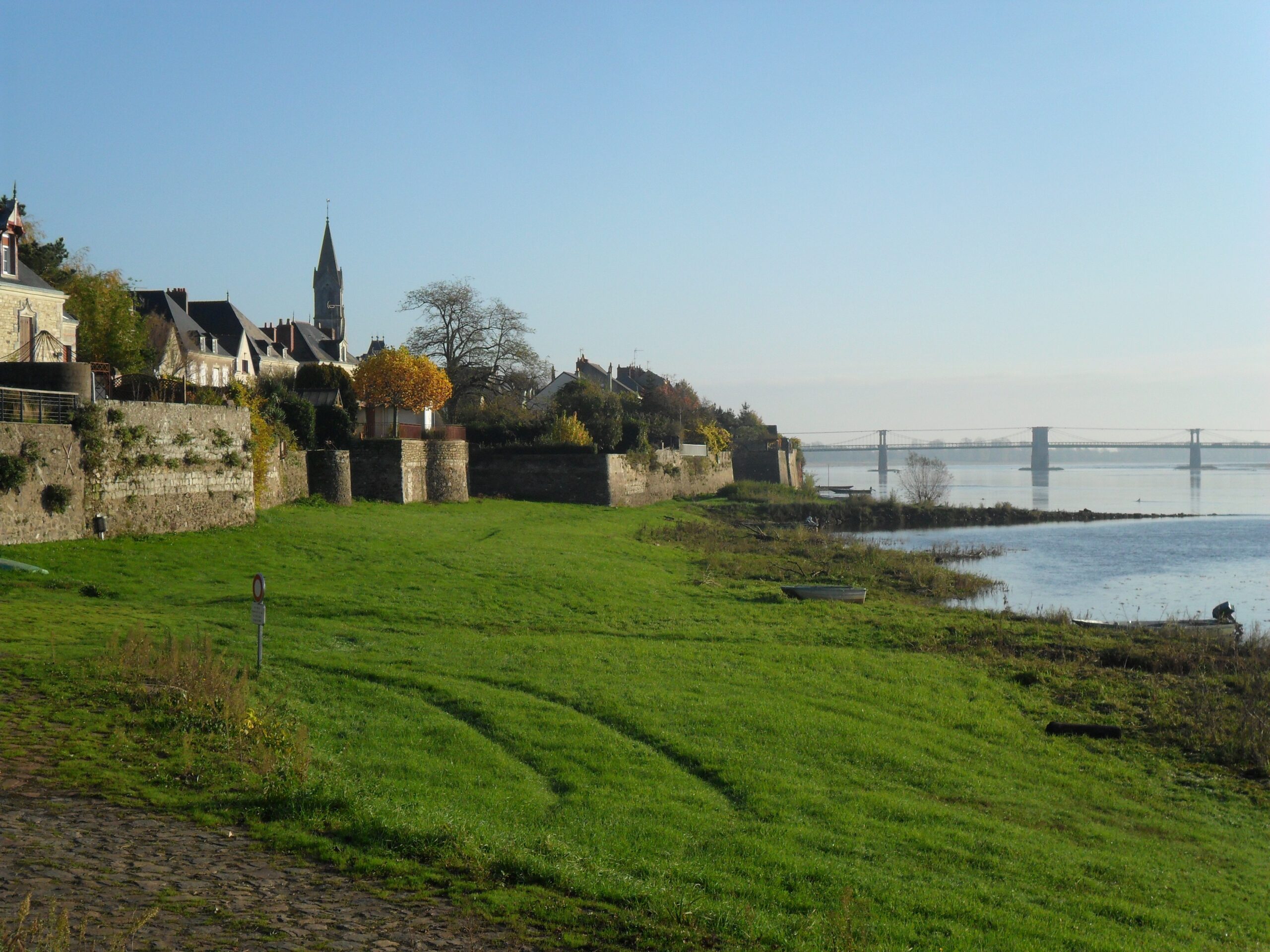
- provision,
- regulation,
- cultural benefits, and
- support (United Nations Millennium Ecosystem Assessment, click here for more information).
Provision
- Water for domestic, agricultural and industrial use. Water from the river Meuse is stored in 80 million m3 storage basins in the Biesbosch inner river delta for 5 months before its final processing into drinking water.
- Fish for food. Millions of salmon once swam in the Rhine and the Meuse, but their numbers declined in the Middle Ages. This was due to the construction of watermills in tributary rivers rather than due to overfishing or pollution (Lenders et al, 2016).
- Mineable resources: hydropower, clay for bricks, and sand and gravel as construction aggregates.
- Military defence lines against invasions. The Netherlands owes much of its independence to its water defence lines, despite occasional failures due to river sedimentation (invasion by army of King Louis XIV in 1672) and river ice (invasion by army of General Pichegru in 1795).
Regulation
- Rivers offer pathways for the discharge of water, ice and sediments.
- Floodplains and connected wetlands form retention zones that attenuate flood waves.
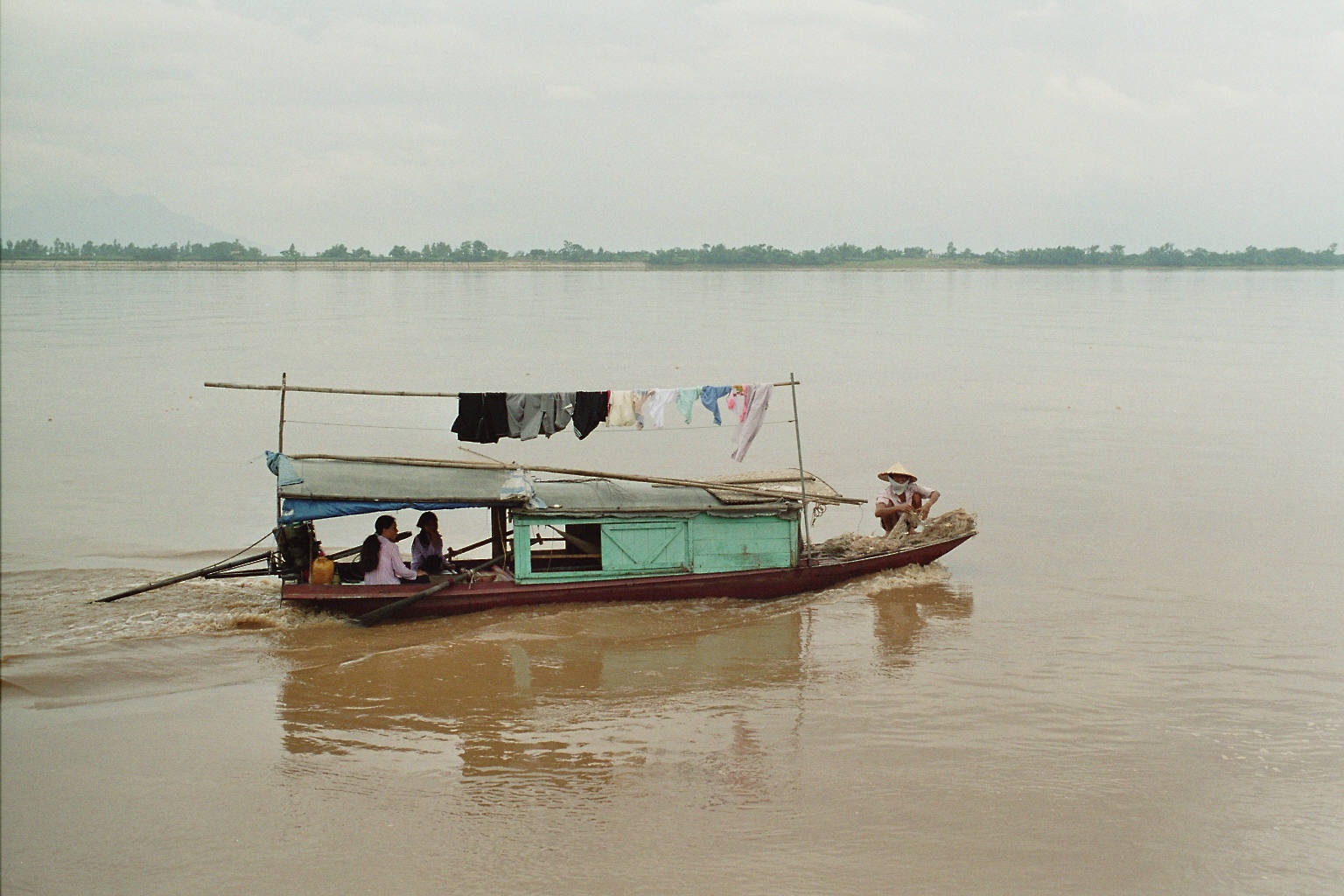
Cultural
- The first civilizations developed on the banks of the Nile, the Euphrates, the Tigris, the Indus and the Yellow River. They left not only archaeological remains but also enduring cultural fabrics with unique identities. Early river engineers founded the first ruling dynasties of those civilizations: Pharaoh Menes or Narmer in Egypt and emperor Yu in China. Renowned philosophers and scientists often worked as river engineers too, such as Thales of Miletus and Leonardo da Vinci.
- Rivers have spiritual values. Many saw them as divine entities. For millions of Hindus, the Ganges River remains the mother goddess Ganga Ma.
- Rivers inspired generations of writers (e.g. Mark Twain’s “Life on the Mississippi”), painters (from Aelbert Cuyp’s riverscapes to the impressionist’s views on the Seine), musicians (from “An der schönen blauen Donau” of Johann Strauss to “The River” of Bruce Springsteen), philosophers and poets. Heraclitus used rivers as a metaphor for the passage of time. Many Dutch can recite the first lines of Hendrik Marsman’s poem “Herinnering aan Holland” which glorifies the manmade landscape of the rivers in the Netherlands.
- Rivers have high aesthetic values. The beauty of the forms produced by natural morphodynamics can be appreciated from, for instance, a time-lapse animation of satellite images of the Ucayali River at Pucallpa (http://world.time.com/timelapse/). Manmade beauty is seen in the river fronts of historical cities such as Dordrecht, Deventer and Kampen.
- The historical, spiritual, artistic and aesthetic values make rivers the backbone of cultural heritage and identity. Rotterdam and Shanghai derive their iconic identities from the rivers that run through them.
- Rivers provide a variety of recreational benefits such as swimming, boating, canoeing, rafting, fishing, and strolling along river banks.
- Rivers are attractive tourist destinations, offering services from river cruises to campsites on river banks.
- Rivers are a key object of research and education in geology, geography, hydrology, hydrodynamics, morphodynamics, ecology, logistics and other fields of science and technology.
Support
- Sediment transport to maintain flood conveyance, navigability and habitats
- Habitat provision for plants and animals.
- Seed transport for riparian vegetation.
Building with Nature opportunities
Building with Nature involves measures or interventions that optimize the use of ecosystem services to achieve new functionality, sustainability and new opportunities for nature. All over the world, rivers face challenges for which Building with Nature could offer solutions. Many rivers have lost space in the past by interventions to reclaim agricultural land, to avoid ice jams, to eradicate malaria, to improve navigation and log transport, and to expand urban areas. The resulting confinement increased flood levels and the resulting concentration of flows in the main channel eroded the river bed, often several metres in just one or two centuries. Sediment mining and interruption of natural sediment transport by constructing dams had similar effects (Kondolf, 1997), adding up to the effects of narrowing by river training. The lower water levels resulting from river bed erosion draw down surrounding groundwater levels, drain connected wetlands, and reduce inundation frequencies of floodplains, obliterating valuable riverine habitats and exacerbating the effects of droughts.
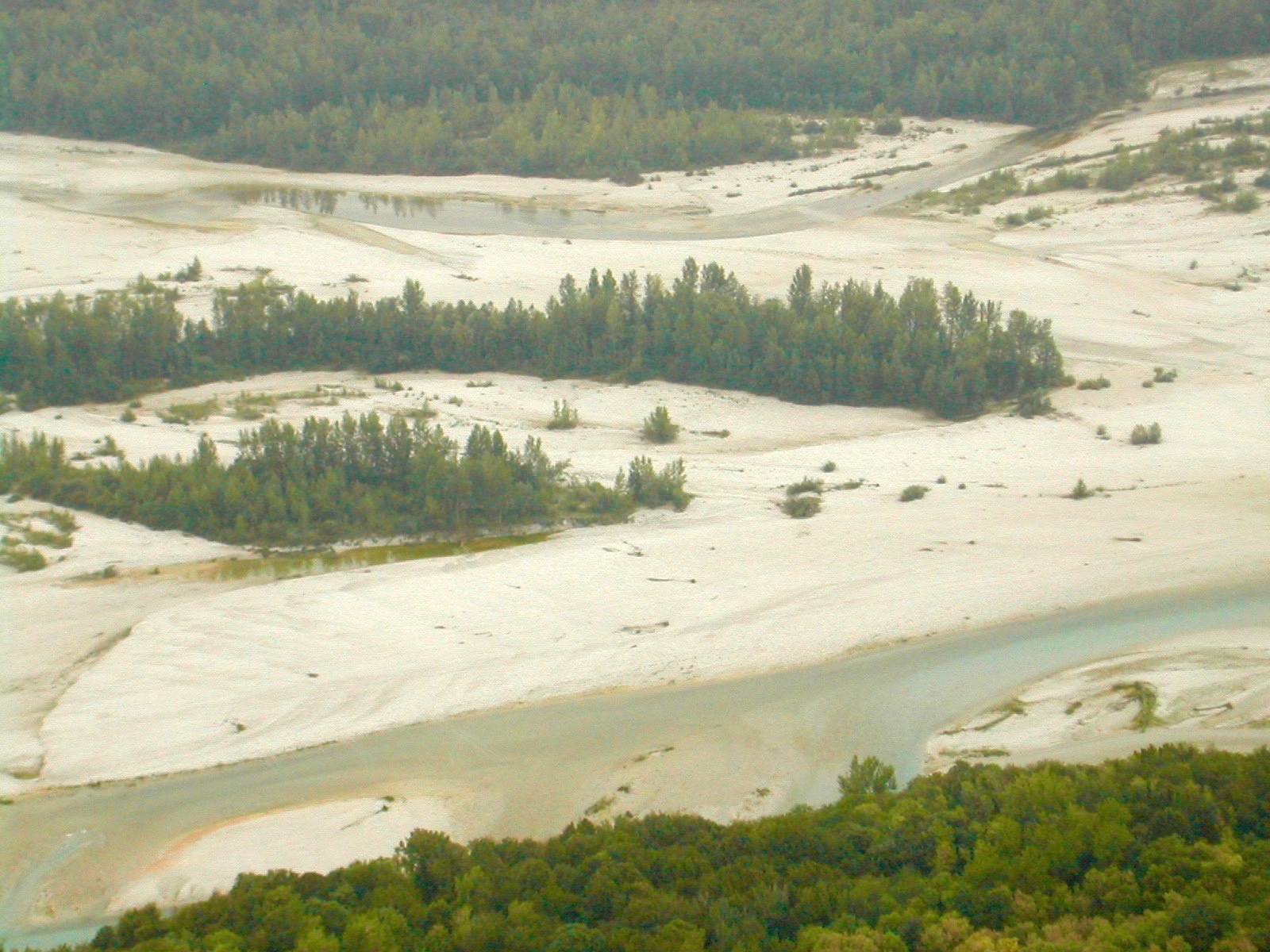
(http://wiki.reformrivers.eu/index.php/Role_of_vegetation)
As the erosion of the river bed is often concentrated in only one channel of a multichannel system, side channels are disconnected from the remaining main channel. Moreover, the erosion threatens the stability of hydraulic structures and produces bottlenecks for navigation at non-erodible parts of the river bed as well as at locations where the fluvial waterway is connected to canals, ship locks and port facilities. Stabilization of the longitudinal river profile has thus become a major challenge in many rivers, including the Dutch Rhine branches (Berkhof et al, 2018). Giving back more space to rivers is one of the solutions to counter the increased flood levels and the adverse effects of bed erosion. This offers major opportunities for Building with Nature. The increased space not only reduces flooding risk and erosion damage; it also creates opportunities for nature and multifunctional use such as use for recreation or cattle farming.
Rivers can be given more space from at least three different perspectives. First, space can be increased to enhance the conveyance capacity of rivers, implying that extreme discharges produce lower water levels. This has been the guiding principle of the Room for the River programme in the Netherlands (Klijn et al, 2018, https://www.ruimtevoorderivier.nl/english/). The added value in this programme was delivered by enhancement of “spatial quality”, an umbrella term for riverine nature, landscape and cultural heritage (Klijn et al, 2013). Second, space can be increased to create retention polders where water is stored temporarily to shave off discharge peaks. The retention polders can develop into ecologically valuable wetlands. This is a key element of the Integrated Rhine Programme in Baden-Württemberg (https://rp.baden-wuerttemberg.de/Themen/WasserBoden/IRP/Rueckhalteraum/irp-en.pdf). An additional benefit of excavating retention polders is that it produces a stock of sediments for nourishment of the eroding Rhine river bed downstream of the dam at Iffezheim (http://www.wsa-freiburg.de/projekte/lsg/index.html – with recommended video). Third, space can be increased to allow natural riverbank erosion and meandering (Piégay et al, 2005). Erosion at one location entails the formation of pioneer landforms by deposition of sediments at other locations. These pioneer landforms provide habitats that sustain cyclic rejuvenation and succession (Peters et al, 2006; Geerling et al, 2013; Gurnell, 2014; Gurnell et al, 2016).
Sediment nourishment is another measure for mitigating bed erosion. It stands out as a form of Building with Nature. In rivers, however, sediment nourishment is more difficult than along coasts. Along coasts no harm is done if more material is nourished than strictly necessary. Moreover, the material will remain available in the targeted area, even after a storm. In rivers, on the contrary, quantities must be constrained as larger volumes would cause problems for navigation and safety against flooding. Nourished material might be washed away to downstream and disappear from the targeted area if an extreme flood occurs. Pilots are still needed to learn more. Such a pilot is currently in preparation for the Niger River in Mali (ABFN & EcoShape, 2017). Another example is the Atchafalaya River Project (https://ewn.el.erdc.dren.mil/pub/Pub_2_Terra_et_Aqua_September2015.pdf) .
Sediment nourishment serves not only the volumetric balance to arrest bed erosion. It may also improve bed sediment composition. Fish, for instance, require clean gravel for spawning. Their eggs and hatchlings hide from predation in the pores between the gravel, but they can be killed if finer sediments clog the pores. Spawning habitats may be created or improved by gravel augmentation.
Rivers in developing countries face socio-economic pressures to be developed in the same way as in developed countries, but the detrimental effects on a long term are now better understood than centuries ago. Modern insights call for less rigid and more adaptive approaches to river training, maintaining sufficient space and adopting approaches based on monitoring and employing natural materials and the energy and forces present in the river. This offers opportunities for Building with Nature.
The aforementioned modern insights include the notion of a system approach. Local problems may have causes far upstream or downstream, requiring analysis on catchment scale (https://www.youtube.com/watch?v=NsfmcNHePiE). Local solutions might be insufficient for solving local problems. On the other hand, relatively small local projects to improve connectivity within a wider ecological network could add value far beyond the strict project area.
A new paradigm has been developed in the framework of a maintenance contract for the river IJssel and the Twentekanaal. This paradigm, called SSRS (https://www.ssrs.info/), encourages the local application of materials harvested in the river, such as wood from clearing floodplain vegetation. This enables the development of innovative river training structures using natural materials. It reduces the CO2 emissions that would result from transporting the materials out and away from the river. This blends well with the guiding principles of Building with Nature.
Last but not least, Principles of Building with Nature are also valuable for natural protections of riverbanks and flood defences. For instance, vegetation in river floodplains can be used to reduce the wave loads on flood defences (http://www.woodsversuswaves.com/) and wave attenuating willow forests at Noordwaard, the Netherlands.
Lessons-learned
Experiences from the past have shown that hard-engineering interventions in rivers may provide local solutions on a short term, but often develop problems on a long term. This underscores the need of smarter, nature-based and more adaptive interventions to optimize fluvial ecosystem services in a sustainable manner. Building with Nature is one of the obvious ways to fulfil this need.
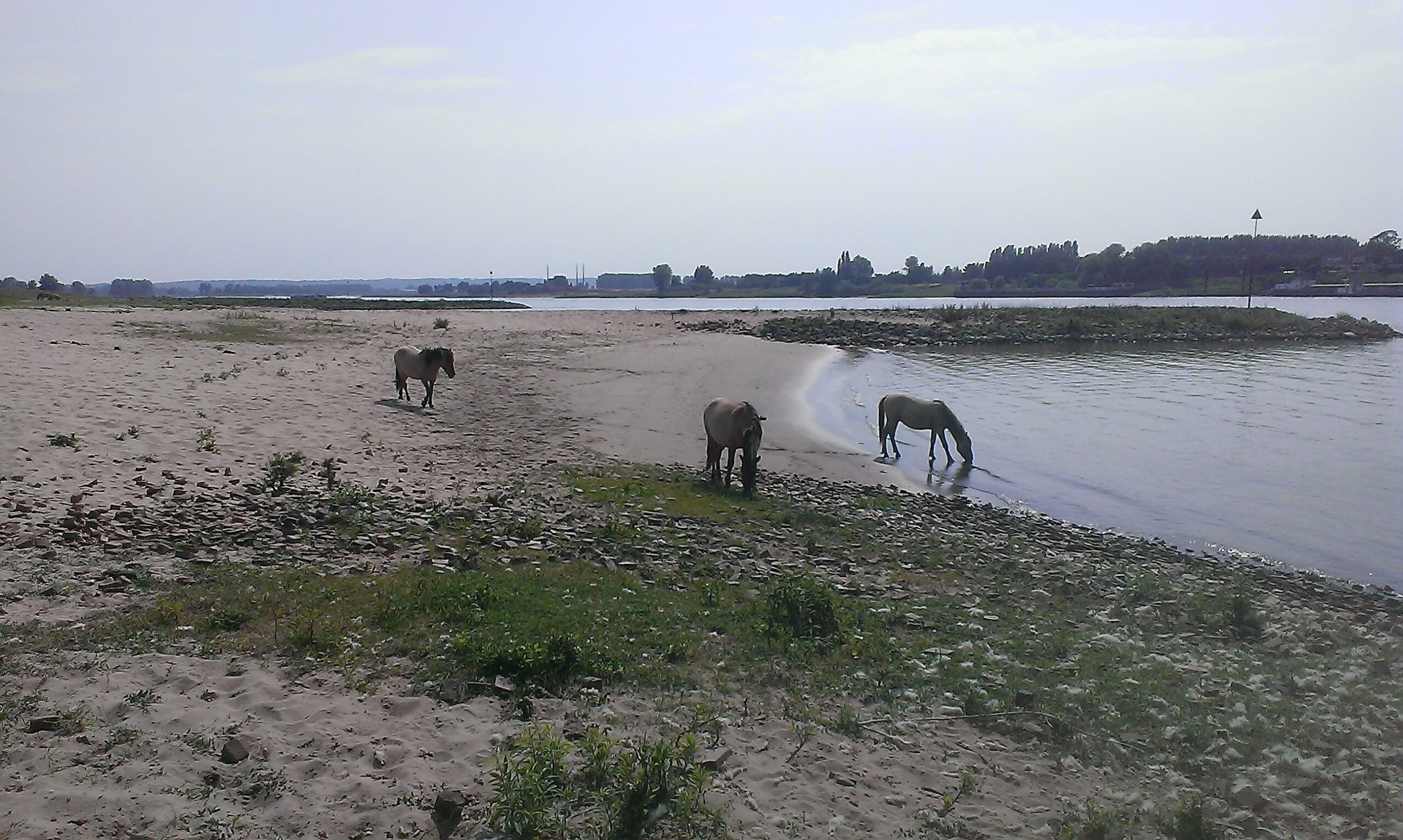
The decline of fluvial ecosystems can often be ascribed to modifications of the physical environment (e.g. Lenders et al, 2016), besides overexploitation and pollution. Building with Nature offers ways to improve or restore the physical environment.
Key interventions in river restoration and adaptive river management are sediment nourishment, targeted vegetation management and giving more space to the river. They all constitute forms of Building with Nature.
Sediment nourishment is more difficult in rivers than along coats. In rivers, requirements of navigability and safety against flooding put a maximum to the amount of sediment nourishment. Unlike extreme storms along the coast, extreme floods on rivers might wash nourished sediments away from the area where they are needed.
Valuable guidance for river restoration is given by the clickable “tiles of wisdom” of the European REFORM project: http://wiki.reformrivers.eu/index.php/Tiles_of_wisdom.
Klijn et al (2017) define the following guiding principles for sustainable integrated river management:
- Evaluate intervention options on the functioning of the system and on minimum regret, taking into account the expected hydrodynamic and morphodynamic response of the system at small and large spatial scales, on a short term and on a long term.
- Keep a buffer space available, to avoid the need of intervening frequently every time when the natural development of the river leads to higher flood levels on the river.
- Respect the spatial differences that are characteristic for different rivers and river reaches, especially when they are relicts from the past and can no longer be created by natural processes.
- Use natural processes in a smart way: soft where possible.
- Combine functions wherever possible, but separate functions clearly when combinations are not possible
References
Literature
ABFN & EcoShape (2017), Programme de restauration des berges et valorisation des plantes aquatiques nuisibles dans le Niger Supérieur. Contribution à la mise en œuvre du Programme de
Sauvegarde du Fleuve Niger au Mali, Agence du Bassin du Fleuve Niger, Bamako, Mali, décembre 2017.
Berkhof, A., J. Kabout, R. Loeve, M. van de Paverd & D. Verhoeven (2018), MIRT onderzoek Duurzame Bodemligging Rijntakken; Eindrapportage, “De Rivierbodem is de basis van alle belangen”. Eindrapport MIRT onderzoek inclusief kostenramingen, Bijlage 1. Arcadis, IenW & Rijkswaterstaat Oost-Nederland, versie 9 mei 2018.
Crosato, A. & M. Samir Saleh (2011), Numerical study on the effects of floodplain vegetation on river planform style. Earth Surface Processes and Landforms, BGRG, Vol.36, No.6, pp.711–720, DOI: 10.1002/esp.2088.
Geerling, G.W., H. Duel, A.D. Buijse & A.J.M. Smits (2013), Ecohydraulics at the landscape scale: Applying the concept of temporal landscape continuity in river restoration using cyclic floodplain rejuvenation. Chapter 23 in Ecohydraulics: An integrated approach, Eds. I. Maddock, A. Harby, P. Kemp & P. Wood, https://doi.org/10.1002/9781118526576.ch23.
Gurnell, A.M. (2014), Plants as river system engineers. Earth Surface Processes and Landforms, BGRG, Vol.39, No.1, pp.4-25, https://doi.org/10.1002/esp.3397.
Gurnell, A.M., D. Corenblit, D. García de Jalón, M. González del Tánago, R.C. Grabowski, M.T. O’Hare & M. Szewczyk (2016), A conceptual model of vegetation–hydrogeomorphology interactions within river corridors. River Research and Applications, Vol.32, No.2, pp.142-163, https://doi.org/10.1002/rra.2928.
Junk, W.J., P.B. Bayley & R.E. Sparks (1989), The flood pulse concept in river-floodplain systems. Can. Spec. Publ. Fish. Aquat. Sci. 106, pp.110-127.
Klijn, F., D. de Bruin, M.C. de Hoog, S. Jansen & D.F. Sijmons (2013), Design quality of room-for-the-river measures in the Netherlands: role and assessment of the quality team (Q-team). International Journal of River Basin Management, Vol.11, No.3, pp.287-299, DOI: 10.1080/15715124.2013.811418.
Klijn, F., W. ten Brinke, N. Asselman & E. Mosselman (2017), Het verhaal van de rivier; Een eerste versie. Deltares en Rijkswaterstaat.
Klijn, F., N. Asselman & D. Wagenaar (2018), Room for Rivers: Risk reduction by enhancing the flood conveyance capacity of the Netherlands’ large rivers. Geosciences, Vol.8, No.224, doi: 10.3390/geosciences8060224.
Kondolf, G.M. (1997), Hungry water: Effects of dams and gravel mining on river channels. Environmental Management, Vol.21, No.4, pp.533-551.
Lenders, H.J.R., T.P.M. Chamuleau, A.J. Hendriks, R.C.G.M. Lauwerier, R.S.E.W. Leuven & W.C.E.P. Verberk (2016), Historical rise of waterpower initiated the collapse of salmon stocks. Scientific Reports, Vol.6, Article number 29269.
Makaske, B. (2001), Anastomosing rivers: a review of their classification, origin and sedimentary products. Earth-Science Reviews, Vol.53, pp.149-196.
Peters, B., E. Kater & G. Geerling (2006), Cyclisch beheer in uiterwaarden: Natuur en veiligheid in praktijk. Centrum voor Water en Samenleving, Radboud Universiteit, Nijmegen, ISBN 10: 90 810586 14.
Piégay, H., S.E. Darby, E. Mosselman & N. Surian (2005), A review of techniques available for delimiting the erodible river corridor: A sustainable approach to managing bank erosion. River Research and Applications, Vol.21, pp.773-789.
Tal, M., K. Gran, A.B. Murray, C. Paola & D.M. Hicks (2004), Riparian vegetation as a primary control on channel characteristics in multi-thread rivers. In: Riparian Vegetation and Fluvial Geomorphology: Hydraulic, Hydrologic, and Geotechnical Interaction, Eds. S.J. Bennett & A. Simon, AGU Monograph, pp.43-58.
Tal, M. & C. Paola (2007), Dynamic single-thread channels maintained by the interaction of flow and vegetation. Geology, Vol.35, No.4, pp.347-350.
Tockner, K., F. Malard & J.V. Ward (2000), An extension of the flood pulse concept. Hydrological processes, Vol.14, Nos.16-17, pp.2861-2883.
Van de Ven, G.P. (1976), Aan de wieg van Rijkswaterstaat; Wordingsgeschiedenis van het Pannerdens Kanaal. Zutphen.
Van Oorschot, M. (2017), Riparian vegetation interacting with river morphology; Modelling long-term ecosystem responses to invasive species, climate change, dams and river restoration. PhD thesis, Utrecht University, Utrecht Studies in Earth Sciences No.141, ISBN 978-90-6266-486-3.
Vannote, R.L., G.W. Minshall, K.W. Cummins, J.R. Sedell & C.E. Cushing (1980), The river continuum concept. Can. J. Fish. Aquat. Sci. 37, pp.130-137.
Ward, J.V. & J.A. Stanford (1983), The serial discontinuity concept of lotic ecosystems. Dynamics of lotic ecosystems, Vol.10, pp.29-42.
Ward, J.V. & J.A. Stanford (1995), The serial discontinuity concept: extending the model to floodplain rivers. Regulated Rivers: Research & Management, Vol.10, pp.159-168.
Wright, N. & A. Crosato (2011), The hydrodynamics and morphodynamics of rivers. In: Treatise on water science, Ed. Peter Wilderer, Vol. 2, Academic Press, Oxford, pp. 135–156.
Internet
http://world.time.com/timelapse/ (beauty of fluvial morphodynamics: enter “Pucallpa” at “Explore the world”, zoom out a bit and travel virtually along the river)
https://www.ruimtevoorderivier.nl/english/ (Room for the River programme in the Netherlands)
http://wiki.reformrivers.eu/index.php/Main_Page (knowledge for river restoration)
http://wiki.reformrivers.eu/index.php/Tiles_of_wisdom (page with clickable guiding principles)
https://www.slideshare.net/Mosselman/river-morphology-asthescienceofsustainability (long-term morphological effects of interventions in rivers)
http://www.wsa-freiburg.de/projekte/lsg/index.html (sediment management in the River Rhine in Germany, presented in the video on “Langfristige Sicherung der Geschiebezugabe“)
https://en.wikipedia.org/wiki/River_Continuum_Concept (river continuum concept)
https://en.wikipedia.org/wiki/Flood_pulse_concept (flood pulse concept)
Toolbox
Delft3D, Open Earth
EO-Riv