Estuaries belong to the world’s most productive natural habitats. They constitute ecologically diverse ecosystems, are home to unique plant and animal communities and have a great intrinsic value. The most common definition applied to the term ‘estuary’ is a semi-enclosed body of water which has an open connection with the sea and in which sea water is measurably diluted with fresh water derived from land drainage (e.g. rivers) (Pritchard, 1967). Estuaries are transitional environments which are subject to both marine (tides, waves, the influx of saline water and sediment) and riverine influences (influx of fresh water and sediment).
The influx of nutrient-rich seawater and freshwater often yields high nutrient levels, both in the water column and in the bed. Moreover, in most of these shallow water environments, the intensity of the physical agents reinforces the connections between benthic (i.e. bed-related) and pelagic (i.e. water column-related) environments by increasing material processing, nutrient cycling and erosion/deposition. This explains the high primary and secondary productivity of these systems. The physical, chemical and biological gradients typically present in estuarine ecosystems lead to a diverse and complex mosaic of habitats.
Each estuary has a unique combination of features derived from its shape, catchment, connection to the sea, tidal regime and exposure. Different typologies are used to classify estuaries. One is based on how they were formed (e.g. coastal plain estuaries, fjords and lagoons), known as their geomorphology. Another typology (Bowden, 1967) starts from the water circulation patterns (e.g. salt wedge and different stages of mixing). Others consider the relative importance of waves and tides, or the large-scale morphology (see Dürr et al., 2011).
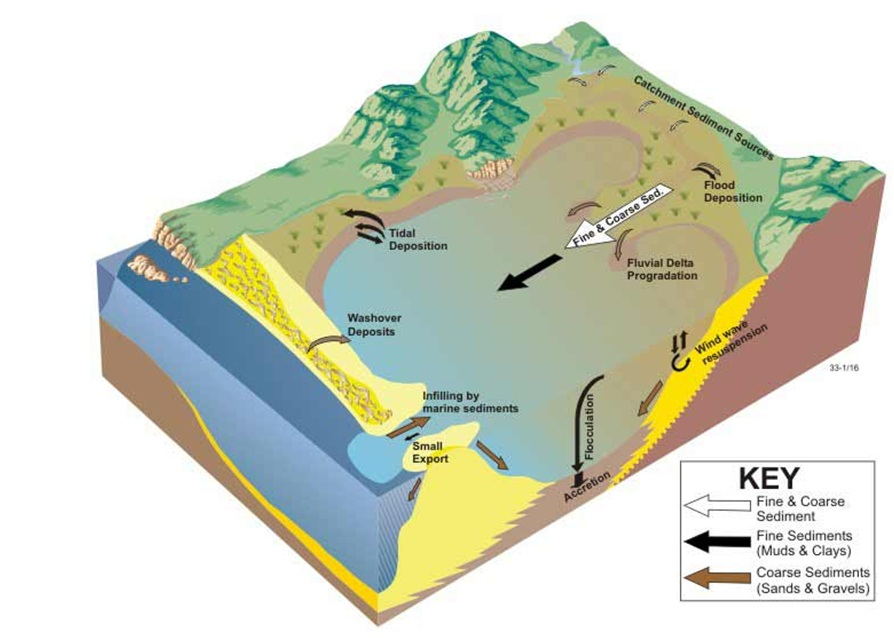
Estuarine ecosystems play an important role in providing food, resting area and breeding grounds for higher trophic levels such as migratory bird species and sea mammals, and they serve as nurseries for multiple fish and crustacean species. They also provide benefits to society, such as food production (e.g. fish, shellfish) and recreation. Further information on human benefits derived from estuaries are discussed in the section Ecosystem services.
Estuaries are susceptible to a number of different stressors (e.g. land reclamation, fisheries, shipping, pollution and sea level rise) that may disturb the natural equilibrium. If this happens, it may have negative consequences for the ecosystem services delivered. Although estuaries provide many valuable ecosystem services (see Barbier, 2011 for an overview), they are among the world’s most heavily used and threatened natural systems. Given the high value of the ecosystem services they provide, it is essential for human activities in estuaries to respect sustainability.
The Building with Nature programme focuses on the case of the Eastern Scheldt (SW Netherlands), a tide-dominated estuary heavily influenced by the construction of a storm surge barrier and compartmentalization dams in the late eighties. Consequently, there is a general trend towards erosion of the intertidal areas. Information and lessons learned from this case may be of use to other estuaries.
System description
Estuaries are very dynamic and transitory systems, influenced by what happens at their landward as well as their seaward end. Estuaries form a complex mixture of many different habitat types which are physically, chemically and biologically interlinked.
Being a transitional area, estuaries are rich in gradients of processes and environmental factors:
• between the hydromorphological dynamics of the river and of the sea
• between fresh river water and saline water
• between river sediment and marine sediment.
These gradients are dynamic, and result in a rich mosaic of habitats with an associated diverse plant and animal life. The transition between river and sea also becomes manifest on a landscape scale: going downstream, the river landscape becomes more open, vast salt marshes with wide flow channels appear, subsequently extensive mudflats or intertidal shoals and finally the sea.
A thorough understanding of the ecology of estuaries requires comprehensive knowledge of the prevailing physical processes and how they interact with the biotic components of the system.
Ecology
Estuaries belong to the world’s most productive natural habitats. The mixture of salt and freshwater present in an estuary challenges the physiology of the animals, only few of which are able to adapt. Freshwater species are becoming less abundant with increasing salinity and are gradually replaced by marine organisms moving up the estuary with some truly estuarine species found at intermediate salinities. Brackish waters in general are assumed to exhibit a species minimum in the oligohaline zone, i.e. the zone with salinities between 0.5 and 5 ppt (Remane & Schlieper, 1971; Ysebaert et al., 2003). A remarkable group of animals in the estuaries are migratory fish, such as salmon and sturgeon. These species can bridge the transition from fresh to salt water and back, under the condition that they have the opportunity to acclimatize in the brackish transition zone.
Besides food, the often sheltered estuarine environment also provides other functions to species, such as breeding-, resting-, nursery grounds. Migratory birds rest and feed in estuarine habitats, which makes estuaries important stop-overs along bird migration routes. Estuaries also provide billions of larvae of zooplankton to coastal waters. Multiple commercially important sea fish and crustaceans use estuaries as nurseries during their juvenile stage. Thus, estuarine habitats are critical to the survival of many marine species.
The combination of varying physical and salinity conditions in estuaries results in a number of unique habitat types, occurring in a pattern that reflects the prevailing physical processes. Each habitat type supports a variety of species. Although estuaries have a high primary and secondary productivity, estuarine communities have a relatively low species diversity compared to those in fresh or fully saline conditions. This is due to the presence of high-amplitude and partly unpredictable stresses, such as salinity conditions, osmotic stress, hydrodynamic stress, which select a limited set of adapted species.
Depending on its geological and climatic location, an estuary may include the following habitat types: salt marshes, mangroves, seagrass meadows, sandy beaches, rocky reefs, mudflats, sand flats, supratidal flats, shellfish beds, channels and tidal pools.
In the Building with Nature program we discuss intertidal habitats in the temperate region in more detail. Such habitats have a high ecological value and are of key importance for the ecosystem functioning and the provision of ecosystem services.
Tidal flats
Mudflats and sand flats are intertidal and sometimes supratidal sediment bodies which emerge in sheltered coastal environments, particularly in meso- and macro-tidal estuaries and coastal embayments. Tidal flat morphology is dynamic at different time scales: on a short scale it is formed by erosion and deposition processes, on intermediate scales by migration of channels and gullies, and on the long time scale by changes in sea level.
Tidal flats constitute an important part of the estuary landscape. In large estuaries they may be several kilometres wide and occupy more than half the total area. They commonly appear in the natural sequence of habitats between subtidal channels and supratidal salt marshes.
Intertidal flats are hotspots of organic matter input, decomposition, mineralization and nutrient cycling. On the frequently flooded flats, an intense exchange takes place between the soil sediments and the overflowing water. Every tidal cycle, intertidal flats are exposed to air and oxygen. They receive carbon inputs from local primary production by microphytobenthos (microscopic benthic algae), deposition of high-quality phytodetritus (organic waste material from decomposing dead plants) and of low-quality organic matter associated with suspended particles settling from the water column (Herman et al., 1999). The organic matter is rapidly incorporated into a complex decomposer food web or buried in the sediment.
Tidal flats are controlled by a small set of key biotic and abiotic processes and there is increasing evidence that biological activity engineers and structures the tidal flat landscape (Widdows & Brinsley, 2002; Paarlberg et al., 2005; Le Hir et al., 2007). The biota responsible for changes in the geomorphology and biogeochemistry of soft substrates are termed ‘ecosystem engineers’ (Jones et al., 1997). They can be divided into two main functional groups, namely ‘biostabilizers’, causing increased sediment stability and a reduced erosion potential, and ‘biodestabilizers’, doing the opposite (Paterson & Black, 1999; Reise, 2002; Widdows & Brinsley, 2002; Bouma et al., 2008; Montserrat et al., 2008). Stabilizing key species of tidal flats are for example microphytobenthos, sea grasses and mussel beds. Benthic macrofauna may have both stabilizing and destabilizing effects.
Biogenic reefs
Tidal flats are often inhabited by epifaunal species, such as oysters, mussels and tube-building worms, which form biogenic reefs. These species are so-called ‘autogenic’ ecosystem engineers, as both their structure and their activity have a strong effect on the environment. Biogenic reefs can have a number of important effects on the environment: they change the near bed water flow and stabilise the substrate, the shells or tubes of the organisms themselves provide a hard substrate for attachment of sessile organisms, and the three-dimensional structure of the reef provides habitat and refuge to other species (e.g. Coen et al., 2007; Borsje et al., 2011). Accumulating faeces, pseudofaeces and other fine sediments provide directly and indirectly an important source of food to other organisms. For these reasons many biogenic reefs have a very rich associated fauna and flora, often much richer and more diverse – at least in terms of macrofauna – than in the surrounding areas (e.g. Bouma et al., 2009; Rabaut et al., 2007; Markert et al., 2009; Troost, 2010). The shellfish reefs are highly productive, provide food and habitat to several (commercially attractive) fish species and improve the water quality by reducing turbidity.
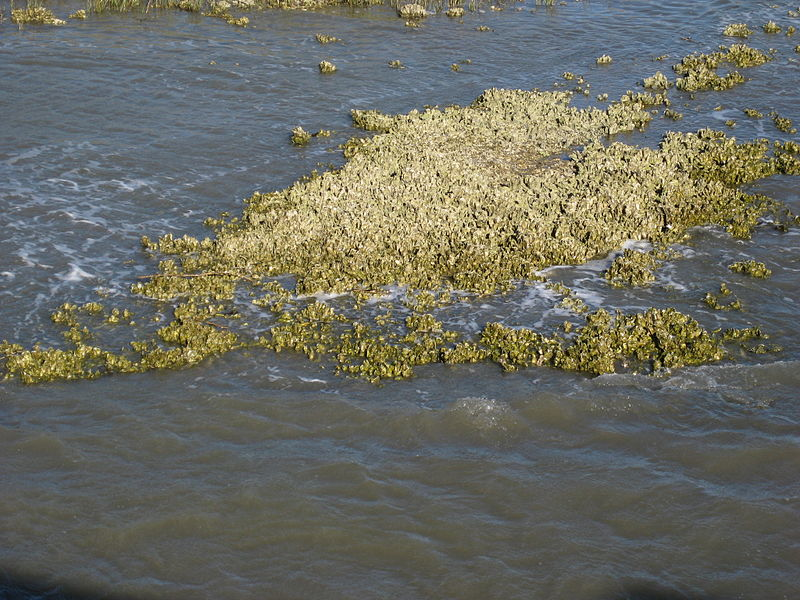
Sea grasses
Sea grass meadows occur in shallow sheltered and soft-bottomed marine coastal zones and estuaries, both intertidal and shallow subtidal. Sea grasses live in the coastal waters of most of the worlds’ continents, both in the temperate zone as in the tropics. A number of environmental parameters are critical to whether sea grass will grow and persist: parameters concerning the physiological activity of sea grass (temperature, salinity, waves, currents, depth, substrate and day length), the natural phenomena that limit its photosynthetic activity (light, nutrients, epiphytes and diseases), and the natural or anthropogenic inputs that inhibit access to available light for growth (nutrient and sediment loading; e.g. van der Heide et al., 2009). Sea grasses provide shelter, food and protection to many organisms, as well as an additional source of organic production and detritus. Sea grasses are generally known as ecosystem engineers. They also reduce flow velocities in their canopies (Bouma et al., 2005). This favours sedimentation, while the roots and rhizomes stabilize the bed (Bos et al., 2007; van der Heide et al., 2007). In temperate North Atlantic estuaries seagrass meadows are dominated by Zostera marina , Zostera noltii and Ruppia maritima .
Tidal marshes
Tidal marshes occur in the intertidal zone, and are flooded part of the time by salty, brackish or fresh water. They are widely distributed in estuarine systems around the world. In temperate and arctic regions they are the equivalent of mangroves in tropical shelf seas and shores. The dominant saline and brackish marsh flora is composed of salt-tolerant plants such as grasses, shrubs and herbs. Tidal marshes occur in low-energy environments that allow for the accumulation of fine sediments and the establishment of plants that are able to survive in a saline environment. Salt marshes often fringe estuaries. Their development is most extensive in estuaries that have a large tidal range, abundant fine-grained sediments, sheltered locations where fine particles can settle and a moderate climate (Allen, 2000).
Marsh vegetation captures sediment, thus causing accretion of its substrate. Thus it becomes less frequently flooded and as a result evolves over time. Pioneer saltmarsh vegetation in Dutch estuaries consists mainly of the perennial common cord grass (Spartina anglica ) and the annual glasswort| (Salicornia spp). Higher up, vegetation communities include among others common saltmarsh grass (Puccinellia maritima) and sea aster (Aster tripolium). In the brackish marshes, seaside bulrush (Scirpus maritimus) and common reed (Phragmites australis) have established apart from Spartina. Final stages of succession include vegetation characterized by Elymus athericus , a wild rye species.
Thanks to their high primary productivity, tidal marshes are used by wintering- and migratory birds and as nurseries by fish. They also play a role in flood defence, as they dissipate wave energy, thus reducing wave attack on adjacent seawalls and dikes (Bouma et al., 2005; Borsje et al., 2011; Shepard et al., 2011). At a larger scale, the provide for flood water storage, thus reducing the penetration of storm surges into the estuary.
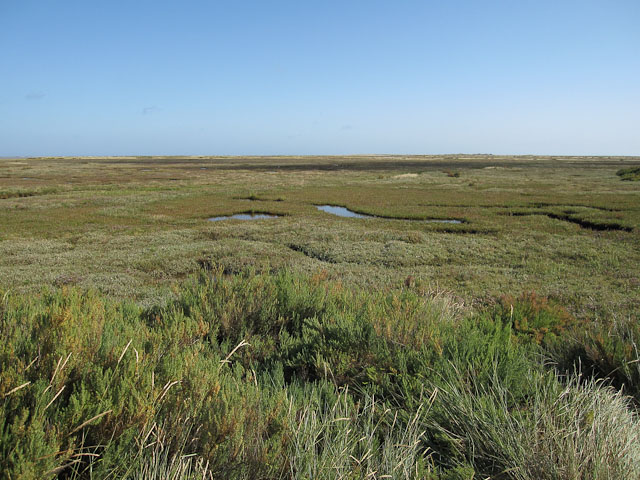
A distinctive feature of an estuary is the nature of the physical dynamics, the result of the interplay of river and sea dynamics. Also its variability within the estuary is characteristic. In the following we describe various physical drivers that play an important role in estuaries.
Hydrodynamics and sediment dynamics
The morfology and ecology of estuaries are largely determined by the hydrodynamics and sediment dynamics of the system. Hydrodynamics are formed by the interplay of tides, river flow and wind generated waves and currents. This interplay results in a wide variety of hydrodynamic conditions in an estuary, yielding a wide variety of sedimentary systems as well.
Tides
Primary physical drivers in estuaries are tides. The predominant tidal frequency may be semi-diurnal (twice a day) or diurnal (once a day). Tides influence salinity and oxygen levels and temperature in the estuary. Strong tidal currents can move sediments such as mud, sand and shells. The volume of water between low and high tide landward of a certain cross-section is known as the tidal prism in that particular cross-section. The tidal prism correlates with the equilibrium cross-sectional area of the channels (O’Brien, 1969). In short tidal basins, such as coastal lagoons, the tidal prism in the mouth also correlates with the total basin volume below the mean water level and the relative intertidal area in the basin.
River flow
Also the influx of fresh river water into an estuary is an important physical driver. The river flow helps maintaining one or more natural outlets to the sea. In areas with a strong seasonal variation of river flows this may even lead to temporary closure of estuaries in times of low flow. Floods and storm events at sea influence the amount of water, sediments and nutrients that are transported from the river into an estuary.
The quantity of fresh river water entering the estuary large determines the salinity distribution in estuarine waters and the degree of vertical stratification. This stratification is caused by the difference in density of fresh and saline water: lighter, fresh water floats on top of heavier saline water. The differences in density hamper the mixing of the water, causing salinity stratification. The degree of stratification determines the extent to which oxygen can reach deep waters and meet the respiratory needs of benthic communities. Also, it determines the degree to which the nutrients released from benthic communities in deep water can reach surface waters and become available for uptake by plants.
Strong tidal currents or wave motions may force mixing and destratification of the water column. The salinity patterns and gradients in an estuary are the result of stratification and mixing processes. These processes also dominate transport and dispersion within estuaries. An informative video on mixing can be found here.
Estuarine salinity gradients are time-varying at a range of scales, associated with the tidal cycle, day-to-day, seasonal and interannual variations in river discharge and tidal range, the occurrence of storm surges, etc. This time-varying salinity is characteristic of estuaries and strongly influences the ecosystem in these areas.
Wind generated waves and currents
Wind in estuaries, tidal basins and lakes not only causes locally generated wave fields, but also non-tidal circulations, especially in areas with significant depth variations. If there is sufficient time and space for the waves to become high enough, they are able to move sediment. Waves tend to ‘shave off’ intertidal flats, down to a level where the transport capacity of the waves has become negligible.
The growth of locally generated wind waves in estuaries is usually limited by the fetch, i.e. the uninterrupted length over which the wind can act on the water surface. Intertidal flats, when emerged, limit the fetch and hence the wave growth. When submerged, the flats limit wave growth by dissipating wave energy in the shallow waters on top of them. In this way, intertidal flats play an important role in the design conditions for the flood defence system bordering the estuary.
Sediment dynamics
The wide variety of hydrodynamic conditions in an estuary also yields a variety of sedimentary systems, from sandy channel beds, via mixed intertidal flats to mudflats and muddy marshlands. Sediment originates from rivers, sea and the land surrounding the estuary (Day et al., 1989; McLusky & Elliott, 2004). Where saline water and sediment-laden river water meet and mix, the increasing salinity may cause sediments suspended in the fresh water to flocculate, resulting in an estuarine turbidity maximum (ETM) and a zone of intense sedimentation. These zones are also known as estuarine entrapment zones or null zones. (see sediment transport models).
A (simplified) conceptual model of shoal formation assumes that the tidal motion tends to build up a shoal, via various asymmetries in the water motion that give rise to a net uphill sediment transport. Waves, on the other hand, tend to erode a shoal, especially its top part. If the equilibrium between tidal and wave action is disturbed, like in the Eastern Scheldt after the construction of the storm surge barrier, this has consequences for the shoal morphology. In the case of the Eastern Scheldt, the waves became more dominant and ‘shaved off’ the tops of the shoals.
Bio-physical interactions
The interplay between physical forces and biota is called bio-physical interaction. Intertidal coastal environments are often inhabited by distinct biota such as sea grasses, salt marsh vegetation and epibenthic oyster reefs and mussel beds. These organisms form three-dimensional structures on and in an otherwise bare soft-sediment environment. These structures interact with the tide- and wave-induced hydrodynamic forces. These reef-building organisms are known as ecosystem engineers, as they are capable to mediate the environment for the entire biological community.
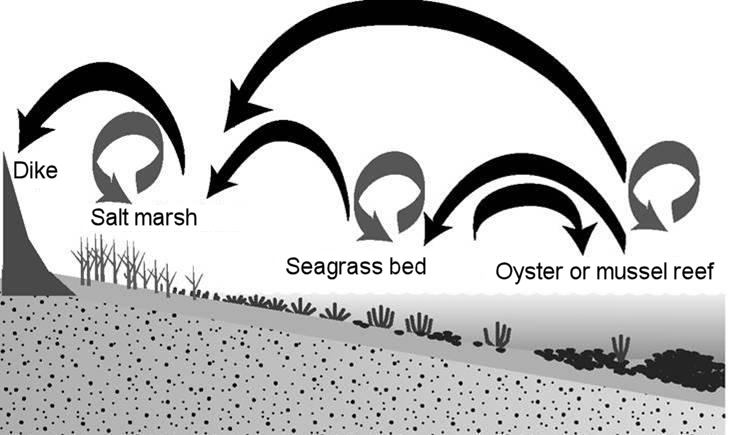
Governance processes
The dominant issue in this environment is coastal safety, but most of the times multiple land use issues also play a role. Therefore, decision making about estuaries often involves national, regional and local governance levels. Furthermore, participation of supranational governance levels may be relevant since activities in an estuary may have their influence upstream.
Traditionally, key stakeholders in estuaries are:
- authorities
- fisheries industry
- environmental organisations
- recreation industry
- local residents (farmers, property owners).
Key actors are national and regional authorities and increasingly also supranational government institutions (e.g. the European Union). In cases where coastal safety is a matter of national importance and solidarity, the national authority is often in the lead, whereas the decentral governments often have an important say in other issues.
Usually, estuarine management has to comply with a variety of development and management plans, sectorial strategies, international regulations – such as EU Directives – and other regional and national policies. Therefore, integration of plans and structure may be a challenge. In Europe, the increasing interest in an integrated approach of estuarine management requires involvement of many parties of more or less equal importance. Stakeholders groups, when confronted with decisions in which they have had no say, will tend to resist against them and they may have extensive legal means to do so.
Although there seems to be consensus on the usefulness of stakeholder participation, public input is often limited in nature and extent. For applying innovative approaches like BwN in estuarine areas with a number of stakeholders groups with the same importance of interests, it is certainly important to actively involve them in the planning process so as to meet their interests as much as possible and minimise the chance of time-consuming court cases.
For more information on governance processes see Governance.
Ecosystem services
Estuaries provide multiple resources and processes from which mankind can benefit. These benefits are known as ecosystem services, commonly divided into four categories (United Nations Millennium Ecosystem Assessment).
- Provision, such as the production of food and water.
- Regulation, such as the control of soil retention.
- Cultural, such as recreational benefits.
- Support, such as nutrient cycles (TEEB).
For further reading on ecosystem services see here.
Provision
Examples of products that are obtained from estuaries are:
- construction materials, such as sand from channel maintenance and wood from mangroves;
- food, such as fish, shellfish, edible halophytes (e.g. glasswort or perennial goosefoot);
- bait. The harvesting of e.g. sandworms for the live sea-bait industry is an economically significant activity.
Regulation
Examples of regulation services of the estuarine ecosystem are:
- water quality regulation, as coastal wetlands capture and filter sediments and organic waste in transit from inland regions to the sea;
- coastal protection against natural hazards and floods by sagrasses, marshes and mangroves that attenuate waves and help to maintain higher foreshores;
- nutrient cycling providing biogeochemical activity, sedimentation and biological productivity;
- erosion control by for example oyster reefs that stabilize sediments;
- flood control, e.g. estuaries and their surrounding wetlands act as buffer zones by storing flood water and protect the riparian areas from flooding;
Cultural services
Examples of non-material benefits people obtain from an estuary are:
- beach tourism and recreation;
- recreational fishing;
- Other recreational activities;
- Aesthetic value (scenic beauty);
- Spiritual and religious values;
- Education.
Support
Services of estuaries which are necessary for the production of all other ecosystem services, but do not yield direct benefits to society:
- Habitat provision for animals and plants;
- Nursery provision such as oyster reefs, seagrass beds, and mangroves;
- Feeding and breeding grounds for different species including migratory species such as birds;
- Soil formation processes (sediment accumulation).
Building with nature opportunities
The BwN sub-programme for estuaries mainly focuses on the protection and the creation of intertidal areas. These areas are of key importance to the estuarine ecosystem and in many estuaries their total size has decreased as a result of erosion after dredging, land reclamation, expansion of harbours, dam and barrier building, etc. Below we present some examples of how estuarine dynamics can be used for sustainable eco-engineering.
Using currents and waves for tidal flat regulation
Within an estuary, currents and locally generated waves determine the erosion, transport and deposition of sediment. The seaward end of the estuary is shaped by erosion and deposition of sediments by wave-driven and tidal currents.
A Building with Nature opportunity is to use the forces of currents and waves to redistribute sediments in the estuary. By redirecting currents and influencing waves by (eco-)engineered structures, erosion or deposition of sediments can be promoted. This can be used to deepen or maintain discharge channels and shipping routes, or to form new intertidal areas. These tidal flats can be a prerequisite for provision, regulating and cultural ecosystem services such as food production, water purification, habitat and aesthetic beauty.
Using seagrass meadows, marshes and mangroves for coastal protection
Wave overtopping and dike instability by undercutting and liquefaction can be reduced or prevented with natural coastal defence ‘structures’ such as shallow foreshores and coastal vegetation. The term ‘coastal vegetation’ may include (a) salt marshes, (b) bottom vegetation plants such as sea grasses and (c) mangroves. These vegetation types attenuate waves, fix the bottom and may even trap sediment, thus helping to protect the coast from natural hazards and floods.
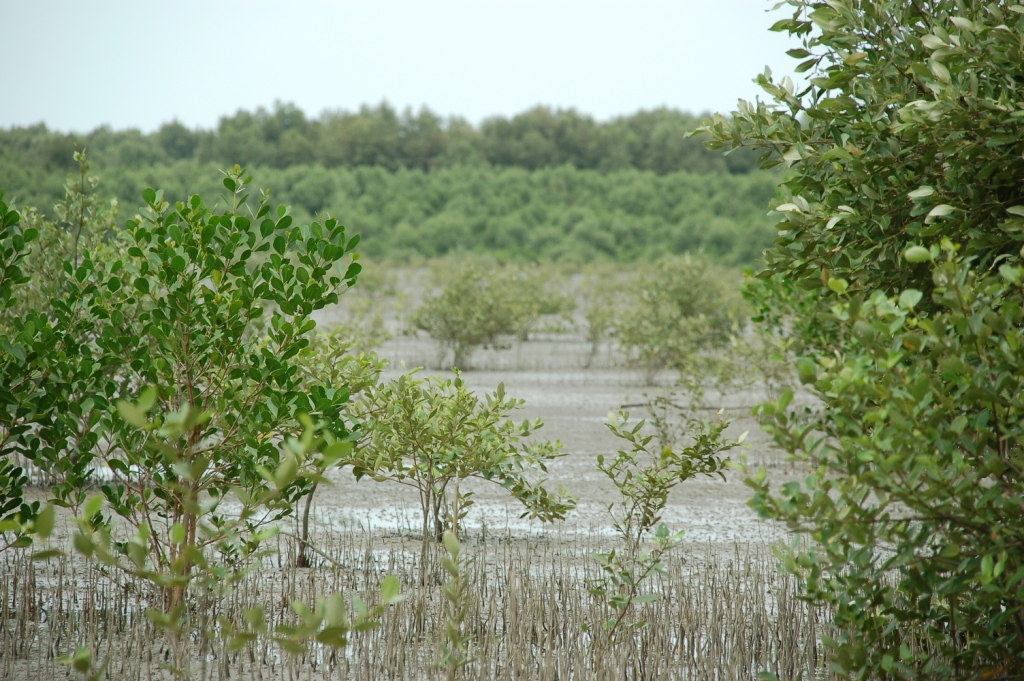
That salt marsh vegetation and mangroves cause significant wave attenuation has been shown by measuring wave height reduction per unit distance across marsh and mangrove vegetation (e.g. Barbier et al., 2008). The effect of salt marsh vegetation on shoreline stabilization has been shown by measurements of accretion, lateral erosion reduction, and marsh surface elevation change (e.g. Borsje et al., 2011, Shepard et al., 2011). Vegetation characteristics that are positively correlated to wave attenuation as well as shoreline stabilization are vegetation density, biomass production, plant characteristics and marsh size. Moreover, these systems have the capability to adapt to gradual variations in environmental conditions, such as climate and sea level.
On top of this, coastal vegetation provide many other ecosystem services. Regulating ecosystem services that are provided are water and sediment regulation, as well as provision services (e.g. wood, food), habitat services and cultural ecosystem services are delivered.
Using ‘ecosystem engineers’ for maintaining or enlarging intertidal areas
Bio-physical interaction by ecosystem engineers can have a strong influence at the local (community) level and have impact on a landscape scale. Reintroducing or promoting these ecosystem engineers can help to maintain or enlarge intertidal areas in the estuary, stabilize shorelines and protect marshes, and provide regulating and supporting ecosystem services (Coen et al., 2007, Borsje et al., 2011, Scyphers et al., 2011) .
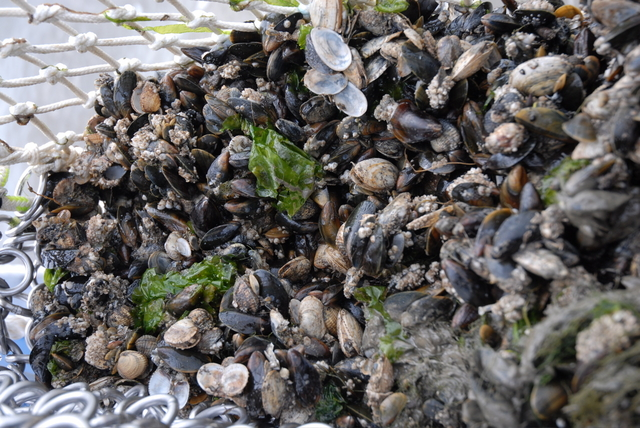
When, for instance, an oyster reef is placed in an estuary, it affects the hydromorphological conditions. This in turn results in wave attenuation and soil formation (regulating ecosystem services) as sediment builds up in the lee of the oyster reef, sometimes creating a shore-protecting sandbank or intertidal flat. Within the accumulated sediment numerous organisms can settle, such as shellfish, worms and other organisms. Such organisms serve as food sources for people (supporting shellfish fishery, a production service), but also for fish (supporting fishery) and birds. The sand banks and fish in their turn may attract seals or otters. The high food availability within the area attracts multiple migratory birds, turning an estuary into a highly valuable foraging and resting area for such birds. The area becomes attractive for bird and wildlife watchers and other visitors (cultural service).
Making revetments ecologically friendly
Dike strengthening while enhancing ecological functions is applied in Case – Eastern Scheldt dike foreshore ecological upgrading. So far, ecological functions have seldom been considered in the design of flood defences. Yet, ecological issues are important. A ‘rich revetment’ is built or enhanced in such a way, that it provides a substrate promoting the settling of filter-feeders (e.g. mussels) and creating habitat diversity. This can be achieved by making new structures more open, or by enriching existing structures by mounting suitable tiles on it. Such measures contributes to the ecological value of a dike and create a net positive contribution to natural value of the dike section. This type of solution can be categorized as Building For Nature.
Creating new tidal areas and habitats by dike realignment
By laying back the primary flood defence and opening up the old one, a new intertidal area is created. This so-called ‘Managed realignment’, developed and applied in the UK, is considered a cost-effective and sustainable response to loss of biodiversity and sea level rise. The goal is to create the right circumstances for succession of tidal flat and saltmarsh vegetation. Once saltmarshes develop the vegetation will enhance sedimentation and the area will become higher and is able to grow with sea level rise. Saltmarshes can reduce wave energy and improve the stability of the dike.
This principle can also be applied to compensate wetlands and (bird) habitat losses caused by e.g. harbour developments. This was done for example at Wallasea, UK, where wetland was created to compensate the impact of harbor development along the British coast. If applied along an estuary or a river, laying back the flood defence also creates extra space for flood water storage, thus helping to reduce the design water levels.
Nourishing intertidal flats
Intertidal flats are valuable habitats for a variety of plants and animals. Worldwide the total area of these flats is decreasing, due to phenomena such as accelerated sea level rise, subsidence by gas extraction or geological mechanisms and erosion initiated by manmade constructions. Nourishing tidal flats may be an adequate mitigating measure, but the impact both on the physical processes and the ecological system are still largely unknown.
Damage to the tidal flat ecosystem, e.g. by burial, cannot be avoided in nourishment projects, but it can be decreased significantly with careful planning. A set of best management practices should be followed, including aspects such as sediment choice, timing, sand placement methods, site-based design, monitoring, and minimizing conflicts of interest.
Lessons learned
So far, the different estuary projects within the Building with Nature programme have taught us a number of lessons. These concern the physical and ecological processes, but also bio-physical interactions and governance.
Physical processes
The physical processes within an estuary need to be well understood, therefore:
- develop a conceptual model of the relevant physical processes (hydrodynamics, sediment transport, salinity intrusion, etc.), and their interactions (morphodynamics at various scales) before undertaking in-depth research into individual processes;
- Make sure that the key processes and their principal interactions at the relevant spatial and temporal scales are well understood, qualitatively and quantitatively. This may include phenomena resulting from long-term interactions of individual short-term processes (emergent behaviour, long-term drift).
Ecological processes
The ecological relations within an estuary also need to be well understood, so:
- develop a conceptual model of the relevant ecological relations and processes, before undertaking in-depth research into individual processes;
- make sure that the role of key species and their principal interactions at the relevant spatial and temporal scales are well understood, qualitatively and quantitatively.
- Gather sufficient knowledge of the ecosystems and the flora and fauna it supports in the vicinity of the project site. Before implementing a measure, be sure to be well-informed of the ecological baseline situation and local physical and ecological dynamics. Also describe the target situation. This situation is not necessarily the same as the baseline situation, as measures may aim at the recovery of a degraded estuary.
- Estuaries are highly dynamic environments. The species in this environment are strongly interlinked, which means that any intervention may affect a multitude of species through their mutual relations.
- Learn from the past. Estuaries are among the most productive, but also most heavily used natural systems in the world. Past interventions and their effects can be used to obtain a better understanding of the system.
- Beware of cumulative effects when working in such an environment.
Bio-physical interactions
When using bio-physical interactions, e.g. when applying oyster reefs or salt marshes (link to ecosystem engineers), be sure that you know the biology and ecology of the key species very well. This is important to decide where, when and how these species can be put to work and what effects can be expected.
Governance
- Conduct a network and stakeholder analysis at the initiation phase. Identify relevant networks, stakeholders and key stakeholders, decide with which networks to align and with which stakeholders to interact, when and how. Especially when scaling up the oyster reef experiments, this helped designing a strategy for collaboration and communication with potentially opposing parties and collaborators. When realizing the shellfish reefs, it turned out to be important to align and start collaboration with parties that:
- Inventory the stakeholders’ perceptions of BwN (and its partners). This provides valuable insight into prejudices and first experiences with the program. People’s perceptions appear to be useful information to open the discussion on future collaboration or improving current cooperation.
- Manage relationships at multiple levels:
- Realise that every experiment has an inherent uncertainty and so has a BwN experiment. Typical of BwN-projects is the uncertainty regarding the long term ecological and / or morphological effects. Be flexible in design, implementation and monitoring of the experiment.
- Clearly communicate the pros and cons of the BwN-approach; provide relevant information openly.
- Realize that laws or regulations, which in the beginning are viewed as barriers, could just as well become an opportunity for development. Usually, this is a matter of interpretation of the law or regulation by the authority responsible for granting the permit.
- Cooperation with environmental organisations appears to facilitate the funding of BwN-initiatives.
References
Literature
- Barbier et al., 2011. The value of estuarine and coastal ecosystem services. Ecological Monographs, 81(2): pp. 169-193
- Bouma TJ, S Olenin, K Reise, T Ysebaert, 2009. Ecosystem engineering and biodiversity in coastal sediments: posing hypotheses. Helgol Mar Res 63: pp. 95-106.
- Costanza et al., 1997. The value of the world’s ecosystem services and natural capital. Nature 387.
- Duffy, J.E. & Smith, W., 2011. J. Emmett Duffy (Lead Author); Walker Smith (Topic Editor) “Marine ecosystem services”. In: Encyclopedia of Earth. Eds. Cutler J. Cleveland (Washington, D.C.: Environmental Information Coalition, National Council for Science and the Environment). First published in the Encyclopedia of Earth October 16, 2006; Last revised Date October 16, 2006; Retrieved September 22, 2011
- van der Heide T., E. Peeters, D.C.R.Hermus, M.M.van Katwijk, J.G.M Roelofs., et al. (2009). Predicting habitat suitability in temperate seagrass ecosystems. Limnology and Oceanography 54: pp. 2018–2024.
- Jones, C.G., J.H. Lawton, M. Shackak, 1997. Ecosystem engineering by organisms: Why semantics matters. Trends in Ecology and Evolution 12, pp. 275.
- Kohsiek, L.H.M., J.P.M. Mulder, T. Louters, F. Berben. 1987. De Oosterschelde naar een nieuw onderwaterlandschap. GWAO 87.029. The Hague: Rijkswaterstaat, Tidal Waters Division (in Dutch).
- Levinton, J., 1995. Bioturbators as ecosystem engineers: control of the sediment fabric, inter-individual interactions, and material fluxes: pp. 29-36. C.B. Jones and J.H. Lawton (eds.) Linking species and ecosystems. Chapman and Hall, New York.
- Pritchard, D.W., 1967. What is an estuary: physical viewpoint. In G.H. Lauff (ed). Estuaries. Washington, DC: American Association for the Advancement of Science.
- Remane, A. and C. Schlieper, 1971. Biology of Brackish Water. New York. J. Wiley and Sons.
- Rhoads, D.C. 1974. Mass properties, stability and ecology of marine muds related to burrowing activity. T.P. Crimes, J.C. Harper (Eds.), Trace Fossils, Geol.J.Spec.Issue 3: pp. 391 – 406.
- Thrush, J.S., J.E. Gray, K.I. Ugland, 2006. Predicting the effects of habitat homogenization on marine biodiversity. Ecol. Appl., 16: pp. 1636-1642.
Images
- Wave dominated sediment transport. From © OzCoasts (Geoscience Australia) 2011
- Tide dominated sediment transport. From © OzCoasts (Geoscience Australia) 2011
- Oyster reef. From Jstuby, 2010. Wikimedia Commons – http://commons.wikimedia.org/wiki/Main_Page
- Salt marshes. Stiffkey Salt Marshes Pools in the upper saltmarsh holding water after the tide has fallen; Hugh Venables, 2009. Wikimedia Commons – http://commons.wikimedia.org/wiki/Main_Page
- Common glasswort. Salicornia europaea, near Southhampton, UK; from Marco Schmidt. Wikimedia Commons – http://commons.wikimedia.org/wiki/Main_Page
- Wildlife watching. Bird watchers at Minsmere near to Eastbridge, Suffolk; From geograph.org.uk (Keith Evans). Wikimedia Commons – http://commons.wikimedia.org/wiki/Main_Page