How to Use
Various steps are needed to undertake when performing a ripening project. This seems like a linear process, however there is a feedback between step4 and step5: monitoring results may request adjustment for the design.
Step 1: Problem analysis and objectives
Before starting a ripening project, it is important to disentangle cause and effect/problem. For example, if your problem is turbidity in an estuary, it is important to understand which causes contribute to increased suspended sediment concentrations. If you solely extract slurries from the system, but navigational fairways are dredged deeper every year, the estuary can maintain its flood dominant state and continues importing fine sediment. As indicated, what is perceived as a problem by one can be viewed as a solution to the other. It is therefore important to take a step back and analyze what is the root cause of the problem in order to intervene in a correct way.
Besides understanding cause and effect it is also worthwhile to state the objectives of the project. Namely, the reasons for consolidation and ripening of clay may vary: solely decreasing turbidity, dredging, land reclamation, generating material for dike construction. Multiple objectives are also possible, nevertheless objectives of the ripening project also prescribe the standards the ripened material should meet. These standards differ per end-product, resources for bricks should have other qualities than material needed to build dikes.
Before using ripened material, users must ensure that the ripened material is ‘sufficiently ripened’ to be harvested and applied in for example dike constructions. Here, ‘ripe enough’ means for example that no cracks will form once applied, or that the material is strong enough to withstand hydrodynamic forces. Parameters that are used to quantify the quality of ripened clay are: 1) erosion class and Atterberg limits which is a function of organic matter and salt content, 2) consistency index, and 3) clay and sand content.
The erosion classes are defined by the Atterberg limits, which consists of two limits of water content (%): the liquid limit and the plastic limit. Respectively, these limits are the minimal water content for which the material starts to flow and the minimal water content for which it is possibly to roll a thread of 3.2mm. The difference between the two limits is the plasticity index (Figure 1). For dike construction, the material needs to have erosion class 1 or 2. Yet, some material has a high liquid limit due to high clay percentages, which means that class 1 is difficult to reach. Salt content changes the clay mineralogy and therefore also the Atterberg limits. Clays consist of silica tetrahedral or octahedral that are stacked with positively charged ions between them. The type of ion can define the type of clay and therefore its properties (Mitchell & Soga, 2005). The ions come from the salt. If clays from a marine environment come into contact with in freshwater and vice versa, the ion compositions in the clay can change and therefore also its Atterberg limits. Salt only leaves sediment by advection; crack formation is therefore important to facilitate drainage trough the sediment. Also, organic content is important which increases the liquid limit as well. This can also be observed in the field, sediment with high organic content is visibly wetter. Organic content leaves the sediment by degradation by microorganisms. This can happen aerobically (carbon dioxide formation) or anaerobically (methane formation), see ’Processes’ section. Crack formation also aerates the soil mediating aerobic degradation, which is the faster trajectory to get rid of the organic matter compared to anaerobic degradation.
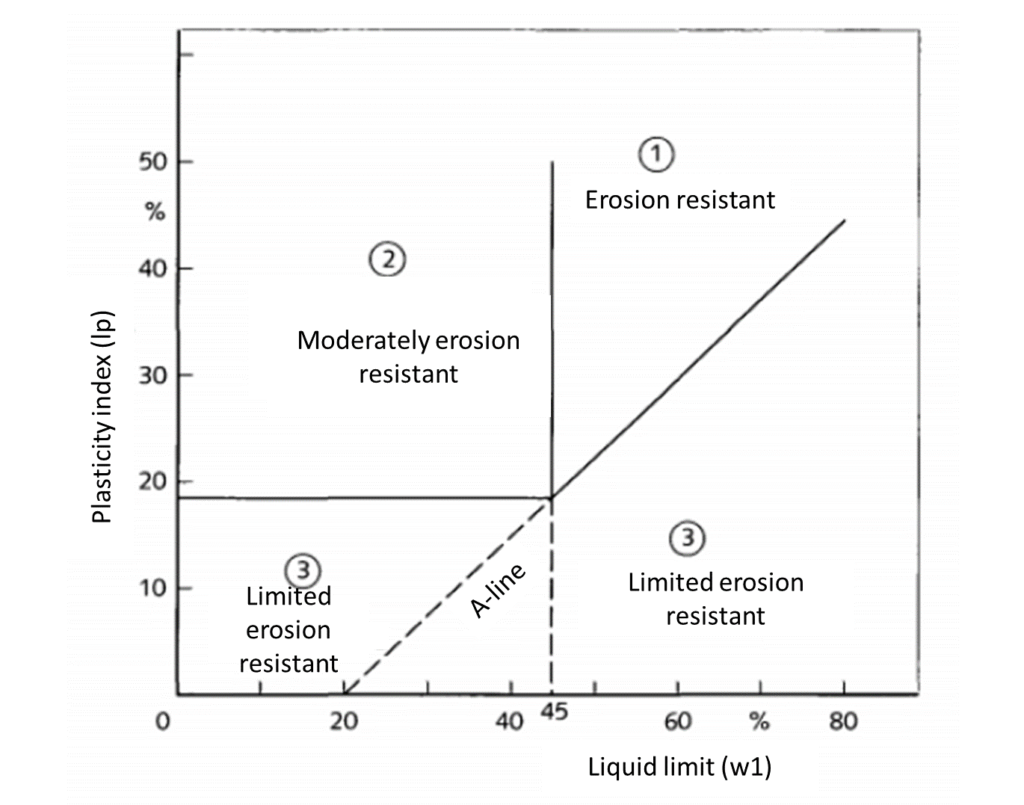
The consistency index is a measure for the water content of clay relative to the liquid and plastic limits. The index is 1 at the liquid limit, 0 at the plastic limit. It indicates the position of the material on its pathway from settling to consolidation and ripening.
Step 2: Natural system analysis and location determination
If the problem, causes and objectives are clear, a system analysis is needed for a comprehensive understanding of the natural, socio-economic and the institutional system. Here we only focus on the natural system. A natural system analysis has a close link with location determination. Because the natural boundary conditions allocate the optimal location for clay extraction and ripening.
Unripe slurries are the base material for ripening and consolidation. Before clay ripening it should be clear what the role is of unripe slurries in the natural system. For example, in a tidal system, slurries are transported bi-directionally by ebb and flood currents but can also accumulate at locations with reduced hydrodynamic conditions like ports. Moreover, in estuaries high suspended sediment concentration can be found at the estuarine turbidity maximum. This is a location at which river discharge and tidal flows interact in such a way that turbidity and the suspended sediment concentration is elevated compared to up- and down estuary (Schubel, 1968). Turbidity has a negative impact on water quality and thus also on biodiversity. Reducing turbidity at these location fosters ecological benefits. A system analysis can shine light on what is the best location to reduce turbidity by extraction of slurries, but also what will be the impact on the system in terms of abiotic and biotic dynamics. Additionally, the sediment characteristic should be assessed. Not only will detailed information on the sediment composition foster decision making of were to extract sediment to meet the ultimate quality standards, also it will give insight into the settling, consolidation and ripening duration. Moreover, investigate whether the sediment contains heavy metals or other pollutants is also essential, because it shapes your ripening location, design and the need to follow certain regulations.
To pick a location for clay ripening some additional points to keep in mind:
- Close proximity to extraction location
- Close proximity to application site
- Elevated to foster drainage
Step 3: Extraction
Once a location is marked from which sediments will be extracted it is important that it is close to an upland ripening location. Since reuse of ‘gebiedseigen grond’ (local material) is a main value of clay ripening and consolidation concept, the extraction location is close to both the ripening location and the final application site. Typically, this can be done by pipes connecting the extraction point with the ripening location (Figure 1 – Overview page).
Noteworthy is that transport trough pipes requires management of the surplus of water that is required for the hydraulic transport. Transport through pipelines also requires management of the surplus of water that is required for the hydraulic transport. Usually this water cannot just enter the water system where the basins are located and require management/return flow. This also accounts for the extruding water during settling, consolidation and ripening.
Step 4: Ripening and consolidation design
The act of ripening and consolidation can be performed in several ways. The methods envelop the natural processes (physical, chemical and biological ripening), but can be catalyzed by mechanical force. Depending on the outcomes of the natural system analysis, a certain design can be chosen.
Groundwater flow
The ripening location can be designed to enhance physical ripening by placing it above the groundwater table so that water can flow downward. Something that can be considered is downward drainage facilitated by horizontal sand layers in a sandwich structure through which the water can flow. Upward water extraction can be mediated by increasing the overburden pressure by capping (placing additional weight on the sediment). Yet, with capping the process at the top of the ripening soil will stop. The process of clay ripening happens mainly at the location where the sediment is in contact with the atmosphere, sunshine, rain, wind and oxygen. The consolidation process helps but should not be leading. Besides, in terms of time, the process at the surface goes much quicker than the consolidation process, which takes a lot of time. Moreover, horizontal dewatering can be facilitated by ditches, 5-15m distance between the ditches is most common (Sjenitzer et al., 2018). Yet, ditches are only possible when the water content of the soil is smaller than the liquid limit, otherwise ditches will collapse instantly.
Turning sediment
Common practice in clay ripening is turning or reworking of sediment over time (Figure 5). The aim of this procedure is to expose unoxidized parts of the clay to the air. This is performed because the effect of ripening is only occurred 30 to 40 cm deep from the exposed topsoil. This means that beneath that level not much is happening. By turning the clay, fresh material is exposed to the atmosphere. Of course, this is only possible once the sediment is strong enough to let heavy equipment surpass the sediment. However, the best method to turn the sediment has been not found yet. Possibly, harvesting the top layer, while leaving the layer underneath as it is, is a more effective method.
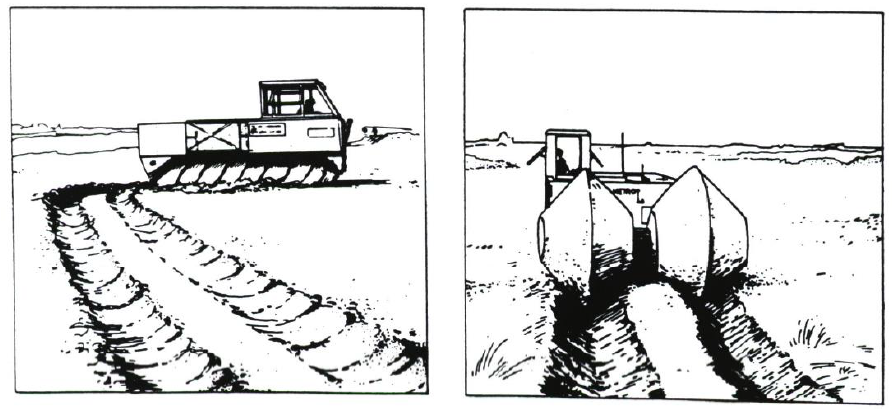
Vegetation
As discussed under ‘processes’ section vegetation can act as and dewatering agent on soils by means of evapotranspiration (Smith, Banks, & Schwab, 2009). The added value of the use of vegetation is that it is also able to survive in- and remove varying pollutants from the soil next to solely ripening (Duggan, 2005; Smith et al., 2009). Yet, because the foliation of vegetation also shades the soil, dewatering by solar radiation is reduced (Sjenitzer et al., 2018). For an estimation of the dewatering potential of vegetation a comparison between a control section and a vegetated part of the ripening location should be performed.
Worms
Also, worms can be used as a dewatering agent as discussed in the ‘processes’ section. In small scale laboratory experiment it has been proven that worms can consolidate sediment a factor 2.5 faster over several days compared to sediment without worms. Worms increase the permeability of the soil by means of making tunnels trough the soil. Large scale application pilots are not applied currently.
Layer height and time
The height of the sediment layer effects the ripening duration. Doubling the layer (Hlayer), means increasing the ripening duration (tripening) with a factor 3. (Geodelft, 1987). Yet, is also depended on the clay and sand content in the soil. Also, the objectives also determine the duration of the clay ripening phase. Material for dike construction have different requirements than for land reclamation. The ambition is to finalize one ripening cycle in one year.
Step 5: Adaptive monitoring
Different criteria are used for different applications for ripened clay. Because dike construction is an important application for ripened clay and is currently under investigation in a pilot called the ‘kleirijperij’, we here focus on dike criteria. Note, these criteria are valid for building optimal functioning dikes. Yet, currently it is under investigation what would be the impact of slight deviations from these criteria on dike performance.
In the Netherlands, the following criteria are valid for clay as a construction material for dikes (Technische Adviescommissie voor de Waterkeringen, 1996):
- The matured clay must at least comply with erosion class II or I. For erosion class II this means: location above the so-called A-line.
- The matured clay must have a consistency index of at least 0.6.
- The sediment must be naturally deposited material.
- Maximum sand content (> 63 μm) of 40%.
- Less than 5% organic matter after hydrogen peroxide treatment method.
- Less than 25% weight loss with the HCl treatment (lime content).
- Salinity (NaCl g / l soil moisture) is less than 4%.
- No significant admixture of rubble, gravel and the like.
- Not much bright (red, brown and yellow, sometimes blue) discoloration.
Adaptive monitoring is needed to check whether the to-be set standards are met within the given time constrain. The ripening process of the slurries to clay is followed through an annual monitoring round in which the parameters for “traditional” dike clay are investigated. Its purpose is to find out whether usable clay (in this case the focus is on dike clay) can be produced from the available saline material in a profitable way (time and effort). The monitoring can be performed intermittently or continuously. For example, soil samples are taken between certain intervals. These samples can reveal the change in soil characteristics (e.g. Atterberg limits, salinity, organic content, water content, ripening index) over time.
The ‘adaptive’ part of monitoring relates to the possibility to adjust the intermission time and the location you sample from, based on previous measurement campaigns. Previous results might evoke a demand for more or less detail over time or distance. For example, over summer much evaporation might take place inducing crack formation, while in late summer a lot of precipitation occurred. Therefore, it would be interesting to check every fall the changes in soil characteristics. However, if the environmental conditions radically change every season affecting the soil characteristics, more frequent measurements should be undertaken.
Continuous measurements can be performed with fiber optic cables that can track the mud-water, the water-air and mud-air interface over time. Fiber optics can also be applied to measure the water content continuously.
Moreover, it is important to monitor the environmental hygiene quality of the prior to filling and must be checked occasionally again in the depots. The groundwater under the depot and the surface water system have to monitored as well to check whether salinity levels remain below the standard.
Step 6: Application
Once the criteria for clay as construction material are met, the ripened clay is transported to the application site. To minimize transportation costs, the ripening site should be close to the application site, see step 2. This is an important consideration, since each time you touch the clay or turn it over, it costs around 0.50 €/m3. Transport is around 5 €/m3 per 10 km, if transported by road anno October 2020.