Hydrosphere (water on or near the earth) – corals
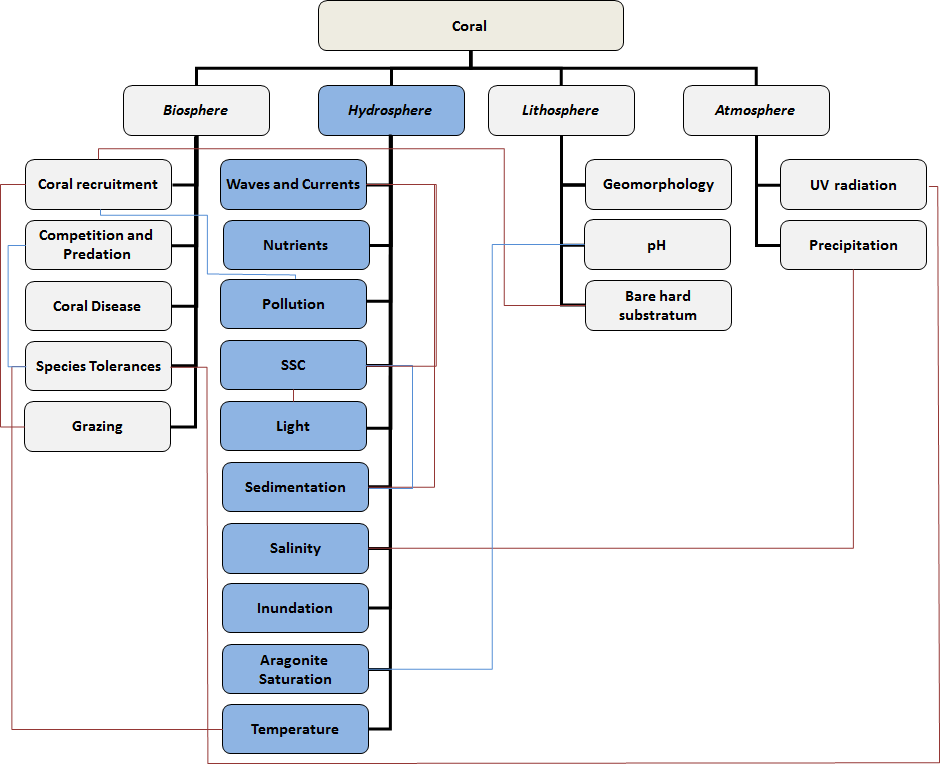
Waves and Currents: “Currents are important in shaping individual reefs and in determining the orientation, shape, and position of the coral sand cays, or ‘low islands’, that develop on reefs. Currents are primarily those generated by the prevailing winds, but in areas where the tidal range is large, tidal effects may become paramount” (taken from Britannica). In addition, currents influence larvae distrubution and therefore the distribution and gene exchange between natural populations. Currents also have large effects on sedimentation (see below). Wave energy has long been recognized as one of the most important factors that control coral growth, reef development and coral island formation. Analysis of a community structure off the west coast of the island of Hawaii shows that variation of wave energy and storm frequency clearly affects the species present in a coral reef as the corals are less prone to breaking when lower wave energy is present (Dollar 1981). Wave energy influences the growth, form and skeletal strength of coral taxa.
Nutrients: Coral flourishes in nutrient-poor environments. Too high a nutrient level can result in a microalgae cover if grazing reef herbivores cannot keep up. Another impact of eutrophication can be higher turbidity due to phytoplankton blooms that will reduce light availability for corals. Nutrient levels (generally ranging from 0.5-5.61 µmol/L for NO3 and 0.1-1.2 µmol/L for PO4) not only increase the growth rate of microalgae, but they may also have direct toxic effects and reduce coral fertility.
Pollution: There are different forms of pollution that may impact a coral reef. Land-based sources of pollution include agriculture, deforestation, harbours, tourism and oil and chemical spills. These sources can result in a variety of impacts including the influx of increased amounts of nutrients, toxins and pathogens. In addition, other sea-based sources such as oil spills, chemical pollution (for example due to chemical industries) or cyanide fishing may have large impacts on the coral reefs. Cyanide fishing, which involves spraying or dumping cyanide onto reefs to stun and capture live fish, also kills coral polyps, and degrades the reef habitat. Pollutants and related synergistic effects can cause disease and mortality for coral species but also disrupt the reef’s ecosystem, having a secondary effect on the corals themselves. Disruption of critical ecological functions can cause a change in trophic structure and by changing the dynamics in the system, impede growth, reproduction and larval settlement. More information can be found on the NOAA coral reef conservation program.
Suspended sediments: Sediment suspension can be caused by natural stirring of bed sediment by currents and waves. Other causes are bioturbation and human activities such as dredging (see this case on reducing the impact of dredging on corals). Suspended sediment can also enter the system via surface runoff via rivers and streams. Suspended sediments impede light penetration through the water column and can also interfere with active feeding of corals. Additional negative impacts on the energy budget arise from active rejection of settled sediments by certain types of coral as for example the mushroom coral (see sedimentation).
Light: Light is a critical requirement for coral growth and persistence, although some taxa are specialized to mesophotic conditions. The zooxanthellae use sunlight to photosynthesise. Without light the photosynthetic rate is reduced and with it the coral’s overall energy budget, needed to fulfill functions including reproduction. Generally, light is thought to be limiting to reef development at levels below 250 μE/m²/s, although growth of individual species may be limited at levels as high as 450 μE/m²/s. In the tropics, surface irradiance is sufficient. However at depth, light is primarily limited through attenuation. Light attenuated in the water column can be significantly increased by phytoplankton biomass and suspended particulate matter such as sediments.
Sedimentation: A high sedimentation rate has a direct negative effect on coral due to smothering and burial. Severe impacts on energy budgets of coral are caused by a reduction of available surface area for photosynthesis, or by active sediment rejection. Examples of active sediment rejection are mushroom corals which can extricate by inflating and deflating over a period of 10-20 hours. However, the mushroom coral is not a good species for coastal protection, as it can move. Even low levels of sedimentation, resulting in a ~2 mm thick veneer of sediments on the reef surface, hinders successful recruitment. Maximum sedimentation rates resulting from an isolated event (e.g. a storm) should not exceed circa 250 mg/cm2/day. The resilience to sedimentation depends on species and on the duration of the exposure. An indicative value for longer term survival is approximately 25 mg/cm2/day.
Salinity: Coral reefs are frequently subject to variations in salinity, due to precipitation, storms, freshwater runoff or periods of prolonged drought. As corals have no mechanisms of osmoregulation, a change in salinity may affect metabolism and survival capacity (Muthiga and Szmant 1987). Optimal salinity environments for coral persistence is between 25 to 40 ppt, although adult forms of some taxa can tolerate short exposures to <10 ppt.
Inundation: While corals can persist at intertidal locations, water temperatures can rise considerably during periods of low water. Exposure to air should be limited to less than 4 hours/day and preferably only during spring tides.
Aragonite saturation: Aragonite is a mineral form of calcium carbonate, CaCO3, and is often used by marine life to form skeletons and shells. The saturation state is the degree to which the aragonite is saturated with a solute. Recent studies show that the calcification rates measured correlate with precipitation rates of inorganic aragonite calculated for the same temperature (Silverman et al. 2007). Globally, the saturation rate is estimated at 3.06-3.8 Ω-arag. This parameter is critical for the creation of the carbonate skeleton for not only corals, but many others like Coccolithophores (extremely abundant and important single-celled algae). Too low values of this parameter can result in skeletons of poor density (less resilient to high hydrodynamic energy), or it may even limit coral distribution. Studies of reef-building corals show a decline in the coral polyps’ ability to create CaCO3 as aragonite saturation levels decrease. Even though the water continues to be supersaturated with the ions necessary to form aragonite, lower ion concentrations have a negative effect on the health of the coral organism. As aragonite saturation levels fall, the organism must divert energy from other important life functions to elevate ion concentrations within the immediate area of the coral tissue to allow it to continue creating its aragonite framework. If the coral polyps cannot produce enough CaCo3, the coral growth will slow down and erosion becomes a threat.
Temperature: Temperature is a critical habitat condition. Corals can exist in water of 20̊ C and in warmer areas, and occur at locations with daily maxima of 36 degrees. However, prolonged exposures to even 1 degree over the desirable temperature can cause bleaching and subsequent mortality. The current rule of thumb is 4 “degree weeks”, which captures both acute and chronic temperature regimes. One ‘degree week’ is equivalent to 1 week of sea surface temperature at 1 degrees above the expected summertime maximum. Two ‘degree weeks’ can indicate either 1 week of 2 degrees above the expected summertime maximum OR 2 weeks of 1 degree above the expected summertime maximum.
Acidity – pH: Acidity influences aragonite saturation, the lower the pH the more energy it costs corals to use calcium carbonate for growth. At some point, if pH is low enough the chemical equilibrium of carbonate in seawater becomes such that uptake of any inorganic carbon is impossible, and the negative effects of this will include poorer photosynthetic efficiency.